Dr Jae Ho Kim | Dr Stephen Brown – Can Tissue Damage Caused by Radiation Treatment Be Reduced?
Radiation therapy is a common treatment for cancerous tumours. However, radiation-induced tissue injury can be a serious side effect of treatment. Dr Jae Ho Kim and Dr Stephen Brown of Henry Ford Hospital, Detroit, USA, have identified molecular processes associated with radiation injury and have developed anti-inflammatory strategies that can prevent, mitigate, and treat tissue damage. By reducing the amount of tissue damage resulting from radiation therapy, physicians may be able to increase the amount of radiation applied to a tumour, allowing for more effective eradication.
In recent years, advances in technology have allowed for the increased effectiveness of radiation therapy for the treatment of cancer. Examples include greater penetration of the radiation dose using high energy x-rays and newer techniques that allow for precision delivery such as intensity modulated radiation therapy and stereotactic radiosurgery and image guided radiotherapy. Despite improvements in tumour targeting, radiation traverses normal tissues and often inadvertently damages healthy tissue surrounding the tumour, fundamentally limiting the radiation dose that can be safely used.
Complementary to technological improvements, medications to alleviate side effects resulting from the treatment have advanced. The working hypothesis of investigators in this field is that if damage to healthy tissue could be prevented or minimised, radiation doses could be increased and allow for more aggressive and effective treatment of tumours. Many classes of drugs have been studied which have potential to enhance the effectiveness of radiation therapy by decreasing normal tissue injury. Dr Jae Ho Kim and Dr Stephen Brown, both of Henry Ford Hospital, Detroit, USA, have shown anti-inflammatory agents are an attractive approach. They have demonstrated that anti-inflammatories both markedly reduce normal tissue injury after radiation exposure and, in addition, sensitize tumours to the damaging effects of radiation. Timing is important. When optimum, anti-inflammatories increase radiation damage to tumours and, at the same time, reduce radiation damage to normal tissues.
Causes of Tissue Damage Following Radiation Therapy
Radiation cell death has been studied for sixty years and was thought to be well understood. The conventional wisdom is that radiation breaks apart, or ionizes, cellular molecules indiscriminately. Most of the cell is composed of water and most ionized water molecules recombine without consequence. Some affected water molecules, although short lived (much less than a second), can travel small distances within cells to react with other molecules.
‘Building on the results of pioneers in anti-inflammatory therapies, we were the first to demonstrate that pan-cytokine inhibition using a small molecule mitigates radiation injury in both acutely responding tissue (i.e. skin) and late responding tissue (i.e. brain).’
However, cell survival is relatively unaffected by the radiation damage to most molecules in the cell; like water, radiation has little consequence on most other cell components such as proteins, lipids, and carbohydrates because of adequate redundancy. In stark contrast, there is only one double stranded copy of DNA in the cell and damage to DNA by radiation can be catastrophic to cell survival. Single strand breaks to DNA are common and repairable because another complementary template exists. Double strand breaks, although rare, result in the death of a cell. Textbooks on radiation biology focus on DNA damage by radiation as the cause of eventual radiation injury. Experimental studies suggest that a number of secondary, less obvious processes can also lead to much greater cell loss and tissue damage than was sustained from the initial damage to DNA.
Most early damage from radiation injury is treatable. Recently, it has become clear that a main contributor to late radiation tissue damage is inflammation. Shortly after radiation exposure to the tissue and organs, large amounts of proteins known as cytokines and chemokines are released from the irradiated cells, signalling to other inflammatory cells in the body to travel to the site of tissue damage for clean-up and repair. The inflammatory cells produce additional cytokines and chemokines. Problems arise when the inflammatory response stays elevated for long periods of time leading to chronic inflammation and tissue injury.
Irradiation, even at low doses, is one of the few factors known to activate the cytokine called TGF-B. TGF-B activates another protein known as the Smad protein, which, when stopped from being synthesised in mice, reduces skin damage after radiation treatment and prevents inflammatory cells from targeting damaged tissue.
One of the key inflammatory cell types are macrophage, cells that are responsible for detecting, engulfing, and destroying dying or dead cells. Macrophages can be beneficial in the early stages of inflammation. They can also cause chronic inflammation and tissue injury, and have been linked to tumour resistance and recurrence following chemo-radiation therapy.
Activated macrophage cause tissue injury after radiation by producing oxidative damage. Under normal conditions, most tissues and organs guard themselves against this type of damage. However, during times of environmental stress, levels of reactive oxygen species (ROS) increase dramatically, overwhelming the cell’s defence systems and resulting in significant damage to cell structures. The generation of these reactive molecules is part of the body’s immune system and functions to rapidly clean wounds from injury. However, the excessive production of ROS can lead to severe tissue damage. Damaging ROS might arise from several sources including infiltrating macrophages. Other cells, such as skin cells, can be stimulated by pro-inflammatory cytokines to produce ROS. Tissue hypoxia (lack of oxygen) resulting from damage to blood vessels is another source of ROS generation.
The roles of the various pro-inflammatory cells and other proteins in the tissue damage processes following radiation treatment remains an area of active study. Researchers Drs Jae Ho Kim and Stephen Brown have devised two approaches to deduce their functions. The first is to suppress cytokine mediated inflammation using small molecule inhibitors to ascertain whether specific macrophages are the major source of the inflammatory cytokines leading to tissue damage.
The second approach of Drs Kim and Brown is to find and eliminate senescent cells (in other words, cells that have ceased to divide) and to determine if their elimination prevents normal tissue injury and if this enhances the efficacy of tumour radiotherapy. This approach is based on their hypothesis that the radiation-induced senescent cells could be the source of release of pro-inflammatory cytokines and chemokines or tissue-damaging proteins. Multiple pharmacological studies are under investigation to remove senescent cells, an area of medicine known as senolytics. The researchers’ preliminary data generated using both these two approaches are highly encouraging, suggesting these are fruitful avenues for future exploration.
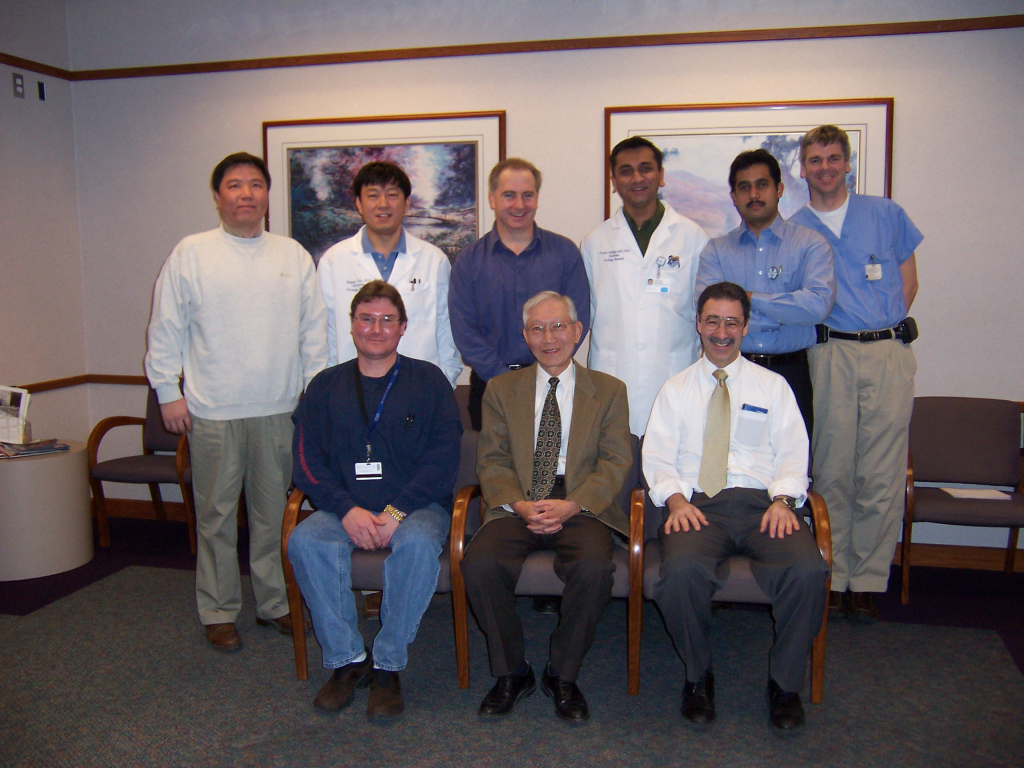
Radiobiology Group in the Department of Radiation Oncology at Henry Ford Hospital
Tissue-specific Radiation Injury
Different tissues can react differently to the effects of radiation. In addition, radiation damage may be classified as acute or chronic, with different molecular mechanisms and cells responses which may be held to blame in each case. The research of Drs Kim and Brown has demonstrated that various tissues targeted by radiation therapy can be affected at the molecular level, and how these different types of damage can best be treated or prevented.
Injuries to the skin as a result of radiation may cover small areas but extend deeply into the soft tissue, even reaching underlying muscle and bone. Symptoms of acute radiation damage to the skin include skin redness and peeling as well as DNA damage. Tissue remodelling due in part to excessive inflammation can occur after radiation exposure. Although the cellular mechanisms remain under investigation, radiation damage to the skin has been attributed to damage to supplying blood vessels and reduced functioning of stem cells that can no longer replace functioning cells.
The research team of Drs Kim and Brown investigated a small molecule known as MW-151, which is a cytokine inhibitor originating from the drug discovery group at Northwestern University. MW-151 was shown to have potential anti-inflammatory properties and promise against brain diseases such as Parkinsons Disease. Drs Kim and Brown found that following skin radiation therapy in mice, MW-151 reduced the damage to tissue, reducing the infiltration of macrophages and the deposition of collagen, which leads to fibrosis (that is, scarring). The effects were highly dependent on the timing of the treatment, with the best results seen on mice given MW-151 on day 7 and day 9 after radiation.
Another organ that can be highly sensitive to radiation treatment-induced injury is the brain. Acute effects occur during and/or shortly after the radiation exposure and are characterised by fatigue and dizziness. From 6 to 12 weeks afterward irradiation, generalised weakness and drowsiness ensue. Although the acute effects are usually temporary, the late effects of brain irradiation may lead to severe irreversible neurological consequences, such as cognitive deficits or death of brain tissue. In the worst cases, seizures and dysfunction of the cranial nerves result from increased intracranial pressure due to fluid build-up in the brain. Of considerable concern is that these effects are usually more pronounced in young children.
The brain has traditionally been regarded as highly resistant to radiation. However, there exist progenitor cell populations in an area of the brain known as the limbic system including the hippocampus, and these are known to be extremely radiosensitive. These cells represent unique sources of new neural cells in the adult brain and are thought to be involved in learning and memory function. When radiation levels are increased in the brain, glial cells known as microglia (similar in function to macrophage in tissues other than brain) become activated and promote the development of chronic neuroinflammation. Anti-inflammatory drugs can reduce microglial activation and chronic neuroinflammation within the brain, partially restoring neurogenesis, or formation of new neurons in the hippocampus. Investigators at Henry Ford Hospital have also used MW-151 to prevent neuroinflammation in rats subjected to brain irradiation and found that this cytokine inhibitor successfully prevented the death of neural progenitor cells and tissue damage when administered to the rats for a period of 28 days.
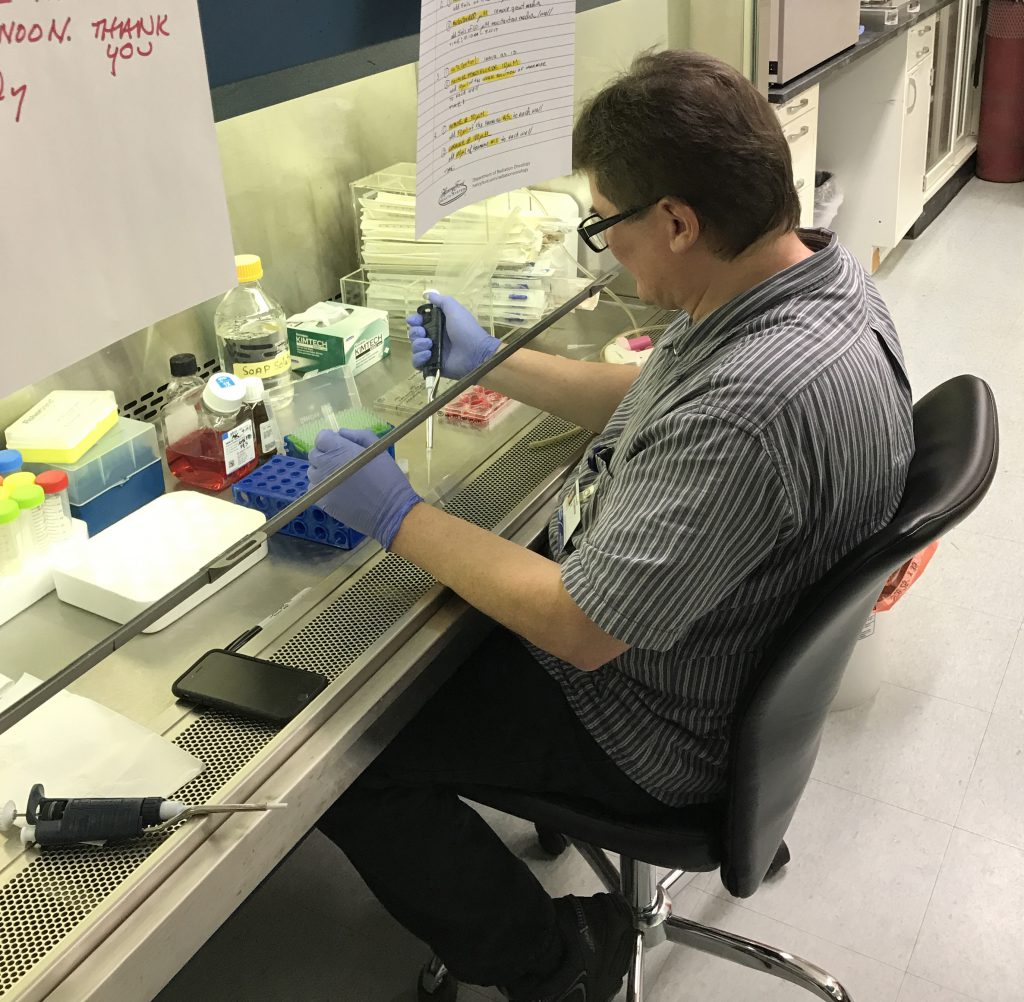
Drs Kim and Brown’s laboratory and Mr Andrew Kolozsvary (Radiobiology researcher).
From the Past to the Future
In summarising their work so far, the researchers explain, ‘Building on the results of pioneers in anti-inflammatory therapies, we were the first to demonstrate that pan-cytokine inhibition using a small molecule mitigates radiation injury in both acutely responding tissue (i.e., skin) and late responding tissue (i.e., brain).’
Obviously, the radiation dose to tumours is critically important, but it is the normal tissue damage which limits the radiation dose that can be applied to tumours. What makes the approach taken by investigators at Henry Ford Hospital unique and different from the previous ones is that their pharmacological approach mitigates radiation injury and damage to the tissues and organs without compromising the effect of radiation on the tumour tissue. In this way, the therapeutic ratio is enhanced. Henry Ford investigators have already identified several drugs which block pro-inflammatory cytokines and ROS that are currently available for other indications and therefore in widespread clinical use.
In conclusion, Drs Kim and Brown have shown that there is value in reducing inflammation, both to normal tissues and tumours after radiation therapy. They continue to seek more effective therapeutic targets that may be used for individuals who are not responding to currently available drugs on the market. Drs Kim and Brown plan to carry out additional investigations to study the cellular- and cytokine-mediated processes underlying radiation-caused tissue damage, particularly in regard to macrophage behaviour. In this way, they hope to discover new molecular pathways that could be targeted for new drugs to prevent or heal radiation-induced tissue damage without compromising tumour control.
Meet the researchers
Jae Ho Kim, MD, PhD, FACR
Department of Radiation Oncology
Henry Ford Hospital
Detroit, MI
USA
Dr Jae Ho Kim is a practising radiation oncologist at the Henry Ford Hospital in Detroit, Michigan, and a Professor of Radiation Oncology at Wayne State University in Michigan. He completed his MD in South Korea at the Kyungpook University School of Medicine in Daegu before completing a PhD in Radiobiology at the University of Iowa in 1963. He then undertook postdoctoral work in Biophysics at the Memorial Sloan-Kettering Cancer Center in New York. Board certified in Radiation Oncology, Dr Kim served as department chair at Henry Ford Hospital from 1989 to 2005. His career has been dedicated to improving radiation therapy for cancer treatment by increasing tumour response as well as by decreasing tissue damage caused by radiation.
CONTACT
Stephen Brown, PhD
Department of Radiation Oncology
Henry Ford Hospital
Detroit, MI
USA
Dr Stephen Brown has been a Senior Staff Scientist in the Department of Radiation Oncology at Henry Ford Hospital in Detroit since 1994, where he specialises in characterising the effects of radiation therapy on human tissue. He completed his PhD in Medical Physics in 1991 at the University of Toronto before moving to Detroit to complete postdoctoral work in the laboratory of Dr Jae Ho Kim at Henry Ford Hospital. His career goal has been to improve the effectiveness of radiation therapy. Since 2009, he has also been a Professor of Radiation Oncology at Wayne State University Medical School in Detroit, and Adjunct Professor of Physics at the University of Windsor in Canada. Since 2014, he has served as Leader of the Translational Oncology Group at the Henry Ford Cancer Institute in Detroit.
CONTACT
W: https://www.henryford.com/physician-directory/b/brown-stephen
KEY COLLABORATORS
Kenneth Jenrow, PhD, Central Michigan University
Andrew Kolozsvary, BS, Henry Ford Hospital
Linda Van Eldik, PhD, University of Kentucky
Martin Watterson, PhD, Northwestern University
FUNDING
NIH R21 CA205660, PI: Jae Ho Kim
NIH R01 CA218596, co-PIs: James Ewing and Stephen Brown