Professor Jonathan M. Kurie – Targeting The Tumour Stroma To Combat Metastatic Lung Cancer
Professor Jonathan M. Kurie and his colleagues at the University of Texas MD Anderson Cancer Center study the mechanisms of lung cancer metastasis for the purpose of identifying novel therapeutic targets. Professor Kurie is specifically interested in understanding how tumour cells control the cellular and extracellular matrix constituents of the tumour and how signals from the microenvironment influence the behaviour of tumour cells.
Lung cancer and metastasis – a deadly combination
When cells break away from a cancerous primary tumour and travel through the bloodstream or through the lymph vessels to other areas of the body this is called metastasis. The metastatic cells that escape from the primary tumour can spread to other organs or tissues in various parts of the body. Lung cancer is the principal cause of cancer-related deaths worldwide. Lung cancer patients primarily die from the metastasis of lung cancer, and not actually from the lung cancer itself. Thus, it is important to understand the biological basis of metastasis in order to develop therapeutic strategies to reduce lung cancer mortality rates.
A tumour cell becomes metastatic in part because of signals from outside of the cell in an area referred to as the tumour stroma. This area consists of cells called cancer-associated fibroblasts (CAFs), inflammatory cells and endothelial cells, all of which secrete molecules known as growth factors, chemokines and cytokines that trigger a complex network of cell-cell interactions within the tumour microenvironment. Professor Kurie’s group believes that targets within the tumour microenvironment are a virtually untapped resource from the standpoint of clinical trial development, and this untapped source of information is a therapeutic research opportunity that might have real clinical benefits for lung cancer patients.
The migrating stem cell hypothesis
In an effort to understand how cancer cells from a lung tumour become metastatic, Professor Kurie and his colleagues focused on the mechanism of the ‘migrating stem cell hypothesis’, a concept proposed and developed by Thomas Brabletz and Robert Weinberg, which is based on the notion that metastases arise from a small population of tumour cells that have the ability to undergo epithelial-mesenchymal transition (EMT). EMT is a reversible process that occurs when cells lose their polarising features, detach from neighbouring cells, become increasingly motile and invasive, and are resistant to standard cytotoxic chemotherapies. There is a factor called ZEB1 that is expressed in lung cancer and is involved in the induction of EMT. ZEB1 represses the genes that could make a cancer cell ‘normal’ and also represses a microRNA family known as miR- 200. The miR-200 family members support normal cell development and suppress the stem cell properties of cells. During EMT, ZEB1 creates tumour cells with stem cell properties, which is a driving factor of metastasis – hence, the migrating stem cell hypothesis.
Environmental cues play an important role in regulating EMT though signals that are transmitted via cell-cell contacts. One of these factors is the Notch axis, which is a system of ligands and receptors that are known to promote EMT, but the mechanism is not clear. Professor Kurie and his team aimed to understand how the Notch signalling axis regulates EMT. Using a lung cancer mouse model they demonstrated that metastatic-prone tumour cells use the Notch ligand Jagged2 to promote EMT by reducing the expression of miR-200. These findings enhance the understanding of migrating stem cell hypothesis and place the Notch pathway upstream of the ZEB1/mir200 axis
The team has shown that in mice that develop metastatic lung cancer, the ZEB1/ miR-200 axis plays a central role in the regulation of metastasis. Based on these data, they proposed that mediators of ZEB1 represent potential therapeutic targets for metastasis suppression. In an effort to address this hypothesis, Professor Kurie and his colleagues examined phosphatidylinositol 3-kinase (PI3K), because this molecule is implicated in the expansion of a variety of normal stem cell populations and tumour-initiating cells in lung cancer models. The findings from their study suggest that ZEB1 activates PI3K in lung cancer cells, and thus makes the mesenchymal tumour cells more sensitive to metastasis suppression using PI3K-targeted therapy. Professor Kurie’s group believes that treatments to selectively transform the metastatic behaviour of mesenchymal tumour cells are reasonable and may have clinical value.
Professor Kurie’s work has further expanded the understanding of the role of ZEB1 in EMT. Transcriptional profiling studies demonstrate that ZEB1 controls the expression of numerous oncogenic and tumour-suppressive microRNAs, including miR-34a. The expression of miR-34a decreases tumour cell invasion and metastasis and inhibits the formation of cytoskeleton structures that promote migration. Professor Kurie and his colleagues found that ZEB1 reduces the expression of miR-34a, which promotes prometastatic actin cytoskeletal remodelling in lung cancer cells. These findings support the development of miR-34a as a therapeutic agent in lung cancer patients and also contribute to the notion that ZEB1 is a key player in the migrating stem cell hypothesis.
‘Apart from trying to kill tumours, one might suppress tumour metastatic activity, which holds promise as an adjuvant to more traditional cytotoxic therapies’
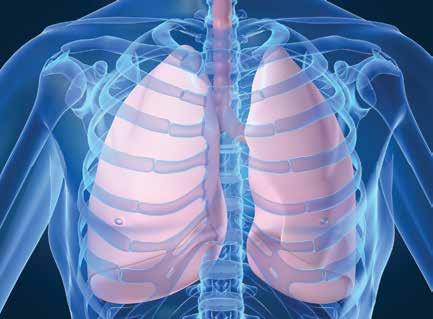
The role of stromal stiffness in metastasis
Within a tissue, the most abundant intrinsic matrix scaffolding protein is collagen. Collagen contributes to the tensile strength of the tissue. Numerous characteristics of collagen metabolism, including its expression, deposition, organisation and turnover, are irregular in lung cancer and are implicated in tumour progression. Tumour extracellular matrix (ECM) stiffness increases when collagen accumulates and is stabilised by the formation of covalent intra- and intermolecular cross-links. Collagen cross-linking occurs in the space outside of the cell by the action of an enzyme called lysyl oxidase (LOX). Tumour cells highly express LOX and LOX-like family members, and this finding led to the development of pharmacological inhibitors of these enzymes. In animal tumour models, the LOX inhibitors reduce the amount of collagen cross-links in the tumours and suppress metastasis, which suggests that the abundance of collagen cross-links in the tumour regulates the disease progression.
Before tumour cells become metastatic there is an accumulation of collagen crosslinks that increases the stromal stiffness and stimulates the invasive properties of the tumour cells, however the biochemical nature of the collagen cross-links in cancer is still unclear. Professor Kurie’s team speculated that the progression of lung cancer is accompanied by changes in the biochemical nature of the collagen crosslinks. In their study, they utilised human lung cancer tissues and a mouse metastatic lung cancer model to show that the tumour stroma has higher levels of hydroxylysine aldehyde–derived collagen cross-links (HLCCs) and lower levels of lysine aldehyde– derived cross-links (LCCs) compared to normal lung tissues. They performed functional studies in the tumour cells and showed that the enzyme lysyl hydroxylase 2 (LH2) shifts the tumour stroma toward a high-HLCC and low-LCC state and increases the tumour stiffness, which enhances tumour cell invasion and metastasis. The data from Professor Kurie’s laboratory indicate that LH2 boosts the metastatic properties of tumour cells and serves as a regulatory switch to influence the relative abundance of biochemically distinct types of the collagen cross-links in the tumour stroma.
Clearly, the stiffness of the tumour microenvironment plays a role in tumour metastasis. This is evident from the development of therapeutic strategies, including inhibitors of LOX and LOX-like family members, which demonstrate antimetastatic activity in preclinical models. Currently, there are no direct inhibitors of LH2 enzymatic activity, but the catalytic activity of LH2 is dependent on the same co-factors (iron, oxygen, ascorbate, and a-ketoglutarate) used by other LHs, and these antagonists already exist and might be modified to selectively inhibit LH2.
Cancer-associated fibroblasts regulate the stiffness switch
Cancer-associated fibroblasts (CAFs) are mesenchymal cells that are morphologically and functionally heterogeneous with diverse origins. CAFs regulate tumour fibrosis, immunosuppression, angiogenesis and metastasis. These unique cells are migratory and contractile and they secrete collagen, cytokines and chemokines into the tumour stroma. Eric Sahai has shown in experimental tumour models that CAFs function as ‘leader cells’ for the invading tumour cells. CAFs lead the group of migrating tumour cells by realigning the impeding collagen fibres to create a path for the invading tumour cells, implying that the realigned collagen fibres within the pathway created by the CAFs have attained stability through the collagen cross-linking. However, it is unclear whether the CAFs actually play a role in regulating the collagen cross-linking.
Professor Kurie and his colleagues wished to address the question of the involvement of the CAFs in collagen crosslinking. Using a lung cancer mouse model, they found that these mice were highly fibrotic and contained CAFs that produced collagen and generated stiffness in collagen gels. In addition, they injected wild-type mice with lung cancer cells alone or in combination with CAFs and found the same total concentration of collagen cross-links in both groups of mice, but the mice that were co-injected with the cancer cells and the CAFs had higher hydroxylysine aldehyde–derived collagen cross-links (HLCC) and lower lysine-aldehyde–derived collagen cross-links (LCCs). Thus, the researchers hypothesised that the LCC-to-HLCC switch induced by the CAFs promotes the migratory and invasive properties of lung cancer cells. To test this hypothesis, Professor Kurie’s group generated co-culture models in which the CAFs were positioned interstitially or peripherally in the tumour cell aggregates in order to mimic the distinct spatial orientations of the CAFs in human lung cancer. The CAFs increased the invasive properties of the tumour cells in both of the coculture models. They also found that LH2 is expressed in the CAFs, and when LH2 is depleted, the ability of the CAFs to promote tumour cell invasion and migration is abrogated.
The Tumour Microenvironment – a bench-to-patient approach
Professor Kurie has established collaborations with a team of scientists at Rice University, the MD Anderson Cancer Center, and the Baylor College of Medicine to integrate cutting-edge technologies from multiple scientific disciplines in order to address the role of the tumour microenvironment in lung cancer progression. This team of scientists plans to follow an approach where the results from the laboratory experiments (at the bench) can be tested in human samples to determine their clinical value (for the patient). The team plans to use these integrated technologies as a platform to identify novel predictive and prognostic factors and therapeutic targets in lung cancer patients. Ultimately, these researchers will incorporate these findings into larger efforts at the MD Anderson Cancer Center and the other institutions to develop personalised targeted therapeutic approaches.
The experimental design is based on three parts. First, Professor Kurie and his team will use a mouse lung cancer model to discover factors secreted by the CAFs that drive lung cancer metastasis. Once they establish candidate factors from the mouse studies, the CAFs and their secreted mediators will be detected immunohistochemically in a large bank of human lung cancer biopsy samples and correlated with clinical outcome.
Secondly, the team will search for the biochemical and physical properties of the tumour ECM that regulate tumour cell polarity and metastasis. After identifying the ECM components using cell culture techniques, they will be detected immunohistochemically in a large bank of human lung cancer biopsy samples and correlated with clinical outcome.
Finally, Professor Kurie’s team plans to examine the interplay between tumour blood vessel formation (angiogenesis), the CAFs and immune cell (macrophage) recruitment, and metastatic invasion. Through these studies they hope to discover the cell-matrix and cell-cell interactions that regulate the rate of angiogenesis as well as the structural properties of the resultant vessels. Once they have identified the pro-angiogenic mediators, the candidate targets will be detected immunohistochemically in a large bank of human lung cancer biopsy samples and correlated with clinical outcome.
Clearly, the common goal of all of these projects is to take data generated from laboratory experiments and test it with actual clinical samples to determine if the findings are meaningful. Through these studies Professor Kurie and his colleagues hope to translate their findings into novel clinical trials that target the tumour microenvironment in lung cancer patients.
Meet the researcher

Professor Jonathan M. Kurie
Department of Thoracic/Head and Neck Medical Oncology
The University of Texas MD Anderson Cancer Center
Houston, TX, USA
Professor Jonathan M. Kurie is a professor the Department of Thoracic/ Head and Neck Medical Oncology at the University of Texas MD Anderson Cancer Center. After obtaining a BA from the University of North Carolina, Professor Kurie received his medical degree from East Carolina University. Subsequently, he completed his residency at the Medical College of Georgia and went on to complete fellowship at the National Institutes of Health and the Memorial Sloan-Kettering Cancer Center. Professor Kurie has been mentoring postdoctoral fellows, graduate students, and technicians and received the Mentor of the Year Award at MD Anderson in 2012. He holds the Elza A. and Ina S. Freeman Endowed Professorship in Lung Cancer and is a member of the American Society for Clinical Investigation.
CONTACT
T: (+1) 713 792 6363
W: http://faculty.mdanderson.org/Jonathan_M_Kurie/
LAB MEMBERS
Marie Wislez
Don Gibbons
Yanan Yang
Young-ho Ahn
Yulong Chen
KEY COLLABORATORS
Chad Creighton, Baylor College of Medicine
Mitsuo Yamauchi, UNC
Ken Scott, Baylor College of Medicine
Jordan Miller, Rice University
Yanzhuang Wang, University of Michigan
FUNDING
National Institutes of Health (R01 CA181184, R01 CA105155, Lung Cancer SPORE Grant P50 CA70907)
Cancer Prevention Research Institute of Texas (Multi-investigator Research Award RP120713)
Lung Cancer Research Foundation
The Elza and Ina A. Shackelford-Freeman Endowed Professor in Lung Cancer Research
REFERENCES
D. Pankova, Y. Chen, M. Terajima, M. J. Schliekelman, B. N. Baird, M. Fahrenholtz, L. Sun, B. J. Gill, T. J. Vadakkan, M. P. Kim, Y.-H. Ahn, J. D. Roybal, X. Liu, E. R. P. Cuentas, J. Rodriguez, I. I. Wistuba, C. J. Creighton, D. L. Gibbons, J. M. Hicks, M. E. Dickinson, J. L. West, K. J. Grande-Allen, S. M. Hanash, M. Yamauchi and J. M. Kurie, Molecular Cancer Research, 2016, 14, 287.
Y. Chen, M. Terajima, Y. Yang, L. Sun, Y.-H. Ahn, D. Pankova, D. S. Puperi, T. Watanabe, M. P. Kim, S. H. Blackmon, J. Rodriguez, H. Liu, C. Behrens, I. I. Wistuba, R. Minelli, K. L. Scott, J. Sanchez-Adams, F. Guilak, D. Pati, N. Thilaganathan, A. R. Burns, C. J. Creighton, E. D. Martinez, T. Zal, K. J. Grande-Allen, M. Yamauchi and J. M. Kurie, The Journal of Clinical Investigation, 2015, 125, 1147.
L. Chen, D. L. Gibbons, S. Goswami, M. A. Cortez, Y.-H. Ahn, L. A. Byers, X. Zhang, X. Yi, D. Dwyer, W. Lin, L. Diao, J. Wang, J. D. Roybal, M. Patel, C. Ungewiss, D. Peng, S. Antonia, M. Mediavilla-Varela, G Robertson, S Jones, M. Suraokar, J. W. Welsh, B. Erez, I. I. Wistuba, L. Chen, D. Peng, S. Wang, S. E. Ullrich, J. V. Heymach, J. M. Kurie and F. X.-F. Qin, Nature Communications, 5, 5241.
Y. Yang, Y.-H. Ahn, Y. Chen, X. Tan, L. Guo, D. L. Gibbons, C. Ungewiss, D. H. Peng, X. Liu, S. H. Lin, N. Thilaganathan, I. I. Wistuba, J. Rodriguez-Canales, G. McLendon, C. J. Creighton and J. M. Kurie, The Journal of Clinical Investigation, 2014, 124, 2696.
Y.-H. Ahn, D. L. Gibbons, D. Chakravarti, C. J. Creighton, Z. H. Rizvi, H. P. Adams, A. Pertsemlidis, P. A. Gregory, J. A. Wright, G. J. Goodall, E. R. Flores and J. M. Kurie, The Journal of Clinical Investigation, 2012, 122, 3170.
