Dr Giovanni Fazio – The Spitzer Space Telescope: Exploring the Infrared Universe
From 2003 until 2020, NASA’s Spitzer Space Telescope provided an unprecedented view of our universe in infrared. One of the most important instruments aboard the telescope was the Infrared Array Camera (IRAC), which was designed and operated by a team led by Dr Giovanni Fazio at the Center for Astrophysics | Harvard & Smithsonian. Across its 16-year run, the camera gave crucial insights into processes ranging from galaxy formation in the ancient universe, to emissions from supermassive black holes. The discoveries enabled by Dr Fazio and his colleagues could soon be instrumental in aiding observations from even more advanced telescopes.
Infrared Radiation
Every part of the electromagnetic spectrum – from radio waves to visible light to x-rays – can tell astronomers something different about our universe. However, perhaps the most interesting part to study is the infrared range.
Lying next to the visible part of the spectrum, infrared waves have longer wavelengths than red light. Although they cannot be seen with human eyes, they can often be observed using optical telescopes. However, infrared radiation also contains an abundance of information that cannot be gathered through visible observations alone.
All the same, infrared observations face a major challenge: as the waves pass through Earth’s atmosphere, they are strongly absorbed by water vapour, making them incredibly difficult to observe from the ground. Fortunately, there is one clear solution to this problem.
Launching the Spitzer Space Telescope
In 2003, NASA’s Spitzer Space Telescope was launched from Cape Canaveral in Florida, and sent into orbit around the Sun – trailing slightly behind the Earth but gradually drifting away from it. From this prime position, the telescope could easily study infrared radiation originating from sources many billions of lightyears away, completely free from the influence of Earth’s atmosphere.
In addition, the spacecraft was equipped with a supply of ultracold helium. Since the detector itself would normally produce its own infrared radiation due to its heat, it would typically introduce noise to its own observations. However, when cooled to −271°C by the helium, Spitzer became well suited to studying wavelengths across the infrared range.
Dubbed ‘NASA’s Great Observatory for Infrared Astronomy’, the Spitzer Space Telescope was principally designed to investigate four major topics: the early universe, giant planets and brown dwarfs, the planet-forming debris disks surrounding newly-formed stars, and the extremely bright regions found at the centres of some galaxies.
To do this, the telescope was fitted with three cutting-edge instruments. The most important of these was an arrangement of four array detectors named the Infrared Array Camera (IRAC), whose design and operation had been spearheaded by Dr Giovanni Fazio of the Center for Astrophysics | Harvard & Smithsonian since 1984.
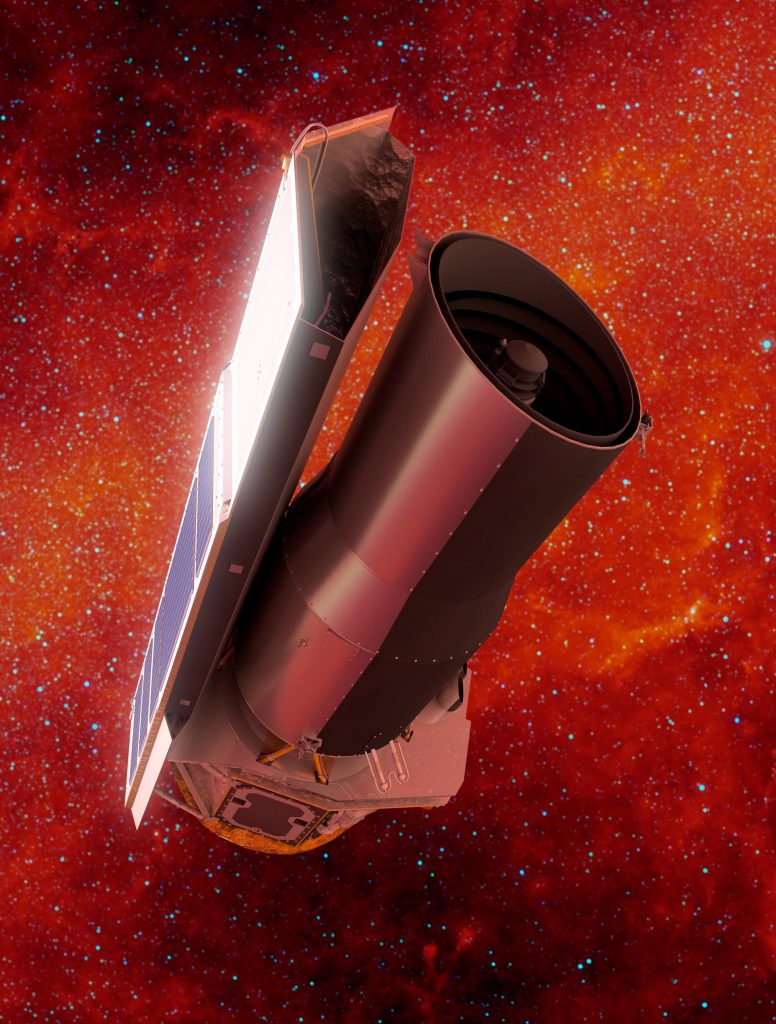
The Spitzer Space Telescope. CREDIT: NASA/JPL-Caltech.
The Infrared Array Camera
At the time of its launch, the IRAC represented a significant leap forward in the capabilities of space-based infrared detectors – boasting high sensitivity, a large field of view, and the ability to produce images in four different infrared wavelengths simultaneously.
For five years after its launch, the instrument operated just as well as Dr Fazio and his colleagues had hoped, with high quality images being produced across the infrared spectrum. As expected, the Spitzer had used up its supply of helium by 2009, and lost its ability to observe longer infrared wavelengths as the spacecraft warmed up.
Fortunately, this didn’t stop the IRAC from picking up shorter infrared wavelengths, closer to the visible range. As a result, the detector could continue operating in full capacity in two of its four wavelength bands until 2020, until Spitzer finally retired after a 16-year run.
Over that time, the IRAC captured striking infrared images of a wide variety of astronomical structures, and led to several important discoveries about their formation and evolution. Dr Fazio was involved in two particularly important branches of this research: exploring the formation and evolution of galaxies in the early universe, and the characteristic emissions from material found at the centre of our own galaxy.
Observing Ancient Galaxies
For much of its first few hundreds of millions of years, the universe was a largely dark, uneventful place, full of hydrogen atoms in their neutral, unionised form. Yet as matter was drawn together under its own gravity, it eventually came to form stars. The intense radiation from these stars stripped the electrons from neutral hydrogen atoms to form hydrogen ions, initiating a new era called ‘reionisation’. Around this time, matter was also coalescing on larger scales to form the first galaxies.
Because of the limited speed of light, the wavelengths emitted by the most distant galaxies we can observe began their journeys many billions of years ago, just as the reionisation era was getting underway. As a result, they can provide astronomers with a clear window into this long-distant period in the history of the universe.
Prior to Spitzer, there was much debate among astronomers as to how early on in the reionisation era that galaxy formation first began. Through infrared observations made by the IRAC, as well as visible and ultraviolet observations from the Hubble Space Telescope, significant strides have been made towards solving this intriguing mystery.

Infrared image of the Helix Nebula taken by NASA’s Spitzer Space Telescope. CREDIT: NASA/JPL-Caltech.
Rethinking Reionisation
In a study published in 2016, measurements taken by both Spitzer and Hubble were used to investigate a galaxy named GN-z11. Positioned more than 13 billion lightyears away from us, this galaxy is one of the oldest known to astronomers – forming around 400 million years after the Big Bang.
Previously, calculations had predicted that reionisation in the early universe must have peaked roughly 550 million years after the Big Bang. Intriguingly, however, these new observations from the IRAC and Hubble showed that GN-z11 had a similar brightness to galaxies observed around the time of this peak, despite being 150 million years older.
This result suggested that the build-up of galaxies could have been well underway shortly after the beginning of reionisation – far earlier in the universe’s history than many astronomers previously thought. If correct, this would suggest the need for a fundamental re-think of how stars and galaxies first formed.
Ultimately, Dr Fazio and his colleagues hope that the IRAC’s observations will now fuel future interest in this mystery. Today, new generations of upcoming space telescopes have built on the capabilities of their predecessors, and could soon lead to discoveries of large numbers of similarly ancient galaxies – potentially transforming our understanding of how they formed and evolved.
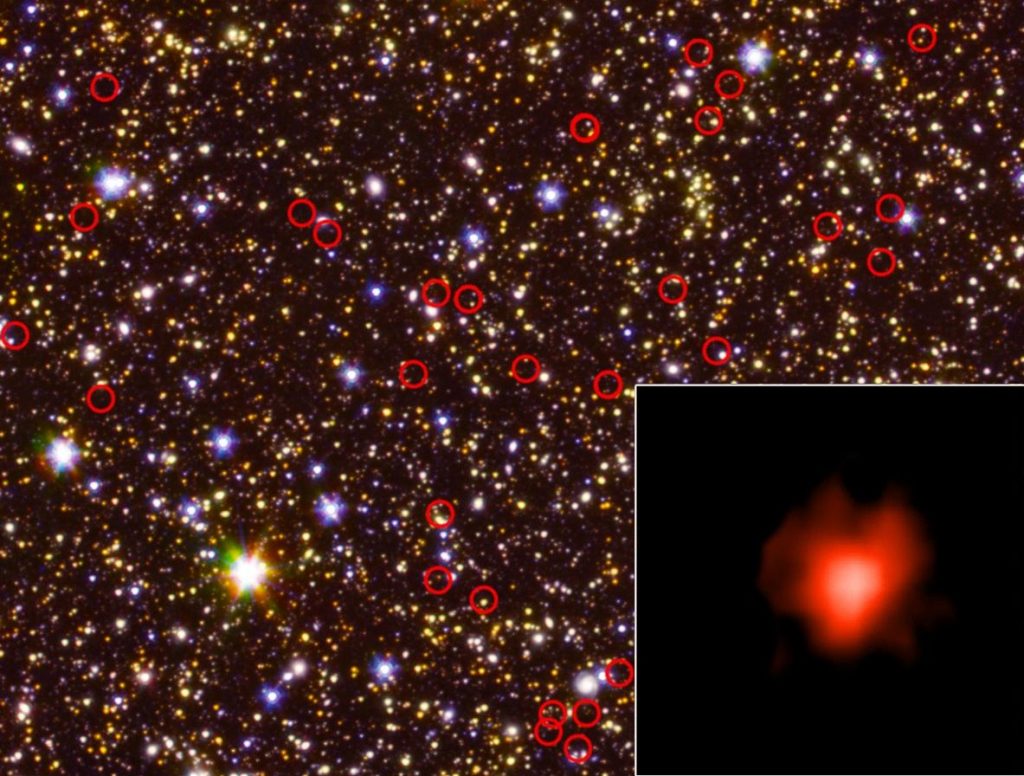
Deep-field view of the sky dominated by galaxies. Very faint, distant galaxies are circled in red. CREDIT: NASA/JPL-Caltech/ESA/Spitzer/P. Oesch/S. De Barros/I.Labbe.
Analysing Sagittarius A*
While most black holes form as massive stars collapse in dramatic supernova explosions, the supermassive black holes found in the centres of many galaxies are far larger still – reaching billions of times the mass of our Sun. At the centre of our own Milky Way galaxy, around 27,000 lightyears away, lies the supermassive black hole Sagittarius A*. Typically, any material surrounding such an enormous body will possess vast amounts of energy, causing it to glow brightly. Unusually, however, Sagittarius A* is extremely dim – emitting roughly 100 million times less radiation than its maximum possible limit.
With a high density of nearby stars creating a bright background to the black hole, astronomers have long seen Sagittarius A* as a compelling and challenging target for observation. In a 2014 study, Dr Fazio’s team used IRAC measurements to study a disk of gas orbiting and falling into the black hole. Such material caused Sagittarius A* to fluctuate in brightness at infrared wavelengths at a rate of about four times in 24 hours. These continuous observations were about twice as long as the fluctuations measured in any previous infrared observations, opening up new possibilities for future studies.
Variability Across the Spectrum
Infrared is far from the only type of radiation emitted by material falling into supermassive black holes. In addition, waves from across the electromagnetic spectrum can be found – from radio waves to x-rays. Building on their observations of the gas cloud orbiting Sagittarius A*, Dr Fazio’s team combined Spitzer’s data with x-ray measurements taken by several other space telescopes to explore the variability of different wavelengths in more depth. In their 2021 study – one of the last to use data gathered by the IRAC – the researchers discovered that x-rays flares emitted by the black hole on short timescales were less variable than its infrared wavelengths.
After analysing the results with advanced mathematical models, the researchers concluded that this difference arose because the processes responsible for infrared and x-ray wavelengths are intrinsically linked. As fluctuating infrared wavelengths interact with electrons within the infalling material, scattering effects related to quantum mechanics will produce distinctive fluctuating x-rays, after a certain time delay.
This discovery was an important step forward in our understanding of the enigmatic conditions surrounding the heaviest single bodies in the universe. However, Dr Fazio and his colleagues hope that the many lines of research initiated by the IRAC’s observations are only just beginning.
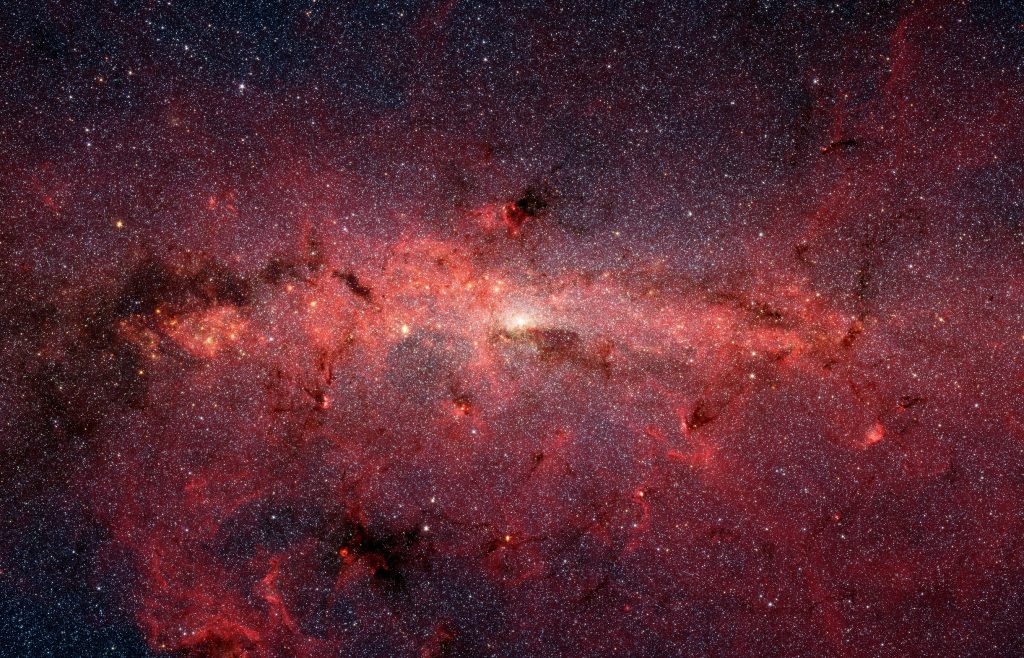
Infrared image of the core of the Milky Way taken by NASA’s Spitzer Space Telescope. CREDIT: NASA/JPL-Caltech.
Informing Future Observations
Throughout its lengthy and highly productive lifetime, the IRAC has led astronomers to draw highly advanced insights into primordial galaxies, supermassive black holes, and a variety of other areas. Now, these researchers are eagerly hoping to continue exploring the infrared universe through a successor to Spitzer: the James Webb Space Telescope, which is scheduled for launch in late-2021.
Encompassing many of the latest advances in technology, the telescope will allow them to characterise the physical properties of these features far more easily. By building on the discoveries of Dr Fazio and his team, their efforts could finally answer some of the most pressing questions about the nature of our universe.
Reference
https://doi.org/10.33548/SCIENTIA688
Meet the researcher
Dr Giovanni Fazio
Centre for Astrophysics
Harvard & Smithsonian
Cambridge, MA
USA
Dr Fazio completed his PhD in Physics at the Massachusetts Institute of Technology (MIT) in 1959. He then moved to the Smithsonian Astrophysical Observatory and the Harvard College Observatory in 1962, and went on to initiate programs including the large balloon-borne telescopes for infrared astronomical observations in the 1970s. Along with his central involvement in the first infrared telescope to fly aboard the Space Shuttle, he also became Principal Investigator for the Infrared Array Camera aboard the Spitzer Space Telescope in 1984, which was launched in 2003, and operated until 2020. Following a highly successful career spanning more than sixty years, Dr Fazio is now a Senior Physicist at the Center for Astrophysics | Harvard & Smithsonian, where his main research interests include development of infrared instrumentation, and observations including ancient galaxies, black holes, and star formation through the use of infrared array cameras.
CONTACT
E: gfazio@cfa.harvard.edu
W: https://lweb.cfa.harvard.edu/~gfazio/
FUNDING
National Aeronautics and Space Administration (NASA)
Smithsonian Institution
This work is based in part on observations made with the Spitzer Space Telescope, which was operated by the Jet Propulsion Laboratory, California Institute of Technology under a contract with NASA.
FURTHER READING
G Witzel, G Martinez, SP Willner, EE Becklin, T Do, A Eckart, GG Fazio, A Ghez, MA Gurwell, D Haggard, R Herrero-Illana, et al., Rapid Variability of Sgr A* across the Electromagnetic Spectrum, 2021, arXiv preprint arXiv:2011.09582.
PA Oesch, G Brammer, PG Van Dokkum, GD Illingworth, RJ Bouwens, I Labbé, M Franx, I Momcheva, MLN Ashby, GG Fazio, V Gonzalez, et al., A remarkably luminous galaxy at z= 11.1 measured with Hubble Space Telescope grism spectroscopy, The Astrophysical Journal, 2016, 819, 129.
JL Hora, G Witzel, MLN Ashby, EE Becklin, S Carey, GG Fazio, A Ghez, J Ingalls, L Meyer, MR Morris, HA Smith, Spitzer/IRAC observations of the variability of Sgr A* and the object G2 at 4.5 μm, The Astrophysical Journal, 2014, 793, 120.
MW Werner, TL Roellig, FJ Low, GH Rieke, M Rieke, WF Hoffmann, E Young, JR Houck, B Brandl, GG Fazio, JL Hora, et al., The Spitzer space telescope mission, The Astrophysical Journal Supplement Series, 2004, 154, 1.
GG Fazio, JL Hora, LE Allen, MLN Ashby, P Barmby, LK Deutsch, JS Huang, S Kleiner, M Marengo, ST Megeath, GJ Melnick, et al., The infrared array camera (IRAC) for the spitzer space telescope, The Astrophysical Journal Supplement Series, 2004, 154, 10.
Want to republish our articles?
We encourage all formats of sharing and republishing of our articles. Whether you want to host on your website, publication or blog, we welcome this. Find out more
Creative Commons Licence
(CC BY 4.0)
This work is licensed under a Creative Commons Attribution 4.0 International License.
What does this mean?
Share: You can copy and redistribute the material in any medium or format
Adapt: You can change, and build upon the material for any purpose, even commercially.
Credit: You must give appropriate credit, provide a link to the license, and indicate if changes were made.
More articles you may like
Dr Michael Parker – Dr Christopher Jeynes | Explaining Fundamental Reality (using the changing entropy)
Dr Michael Parker (Lexden Technologies) and Dr Christopher Jeynes (independent scholar) apply basic principles of thermodynamics to the topical issue of beta-decay bringing us an entirely new understanding of Time and Reality.
Dr Keith Walters | Rebuilding Oyster Reefs with Recycled Shells for Prey Protection
The worldwide loss of coastal oyster reefs negatively affects many organisms that rely on reefs for refuge from predators. Dr Keith Walters and his team at Coastal Carolina University created a series of reefs along the northern South Carolina shoreline using recycled oyster shells from local restaurants. Their investigations of differences in physical characteristics and predator-prey dynamics on newly created and existing natural reefs document that within a year, reef restoration provided a functional habitat for reef-reliant species, quickly reestablishing a key oyster reef ecosystem service.
Dr Sean Kalaycioglu | Beyond the Final Frontier: Controlling Robotics for Space Use
As we explore more of the cosmos, there is an increasing interest in using robotic systems in space, such as rovers, to explore and map out territory or robots to assemble and maintain structures. Dr Sean Kalaycioglu from Toronto Metropolitan University (TMU) and Canadian Space Research Inc. in Canada researches how these systems can be deployed and designed to tackle the unique challenges faced by robots in these environments. Working with his colleagues, Dr Kalaycioglu also develops algorithms and models to optimise the control and performance of these systems.
Dr Barry Ganapol | Discretising Radiation and the Fokker-Planck Equation
Dr Barry Ganapol from the University of Arizona and Dr Ó López Pouso from the University of Santiago de Compostela are working to address the fundamental challenge of solving the Fokker-Planck Equation as applied to radiation transport theory. They introduce a novel application of the Response Matrix/Discrete Ordinates method, achieving six-figure precision in modelling how radiation (specifically electrons and photons) scatters through small angles. This breakthrough could have significant implications for fields ranging from medical imaging to environmental science, enhancing our ability to predict and control radiation’s interaction with matter.