Calculating Steering Friction: Essential Engineering for Military Aircraft Safety
The safe operation of military training aircraft depends on precise engineering calculations that most of us never consider. Dr Bogdan Adrian Nicolin and Dr Ilie Nicolin from the National Institute for Aerospace Research in Romania have developed sophisticated methods to calculate the steering friction moment in military aircraft. This critical engineering parameter ensures that pilots can safely control aircraft during taxiing, take-off, and landing – making their work essential for both pilot training and flight safety.
The Critical Role of Nose Landing Gear
When a military training aircraft moves along a runway, the nose wheel must rotate smoothly to allow the pilot to steer the aircraft effectively. This seemingly simple action involves complex physics and engineering calculations. The friction between the tyre and runway creates resistance that must be overcome by the steering system. Engineers need to calculate this resistance – known as the steering moment of friction – to design appropriate steering actuators that can reliably control the aircraft on the ground. Dr Bogdan Adrian Nicolin and Dr Ilie Nicolin from the National Institute for Aerospace Research in Romania have developed a sophisticated mathematical approach to calculating this crucial parameter. Their work focuses specifically on military training aircraft like the IAR 99NG, where precise steering is essential for pilot training and safety.
Understanding Aircraft Tyres Under Pressure
Before we can understand the steering friction calculations, we need to grasp what happens when an aircraft tyre meets the runway. Unlike car tyres, aircraft tyres experience extreme forces and must withstand tremendous pressure while providing reliable ground handling. When an aircraft’s weight presses down on a tyre, the tyre compresses and flattens where it contacts the ground. This flattening creates what engineers call a ‘contact area’ or ‘footprint’—the portion of the tyre actually touching the runway surface.
For the IAR 99NG military training aircraft studied by the researchers, the minimum vertical load on the nose landing gear during taxiing is 34,335 Newtons – equivalent to the weight of about 3.5 tonnes pressing down on a single wheel. The tyre selected for this aircraft is an Aircraft Rib 20 × 4.4 tyre, which experiences what engineers call ‘tyre deflection’ – the reduction of the tyre’s radius under load. This deflection significantly affects how the tyre interacts with the runway surface.
The Elliptical Footprint
The team explain that when an aircraft tyre is compressed against the runway, the contact area forms an elliptical shape. This elliptical footprint is crucial to understanding how steering forces are distributed. The shape has been confirmed through experimental research on aircraft tyre footprints, proving that theoretical models align with real-world observations.
The elliptical contact area has two key dimensions: its length (2r₁) and its width (2r₂). Within this ellipse, pressure is distributed across the surface where the tyre meets the runway. This pressure distribution, combined with the friction coefficient between the tyre and runway, determines how much resistance the steering system must overcome.
The Mathematical Challenge of Friction
Calculating the steering moment of friction requires sophisticated mathematical modelling. The steering moment represents the rotational resistance that opposes the turning action of the nose wheel. It’s essential that engineers can calculate this accurately to ensure the steering actuators are powerful enough to overcome this resistance in all conditions.
The researchers have developed a precise analytical method for this calculation that accounts for the complex physics involved. The analytical approach uses mathematical integration to sum up all the tiny forces acting across the entire contact area between the tyre and runway.
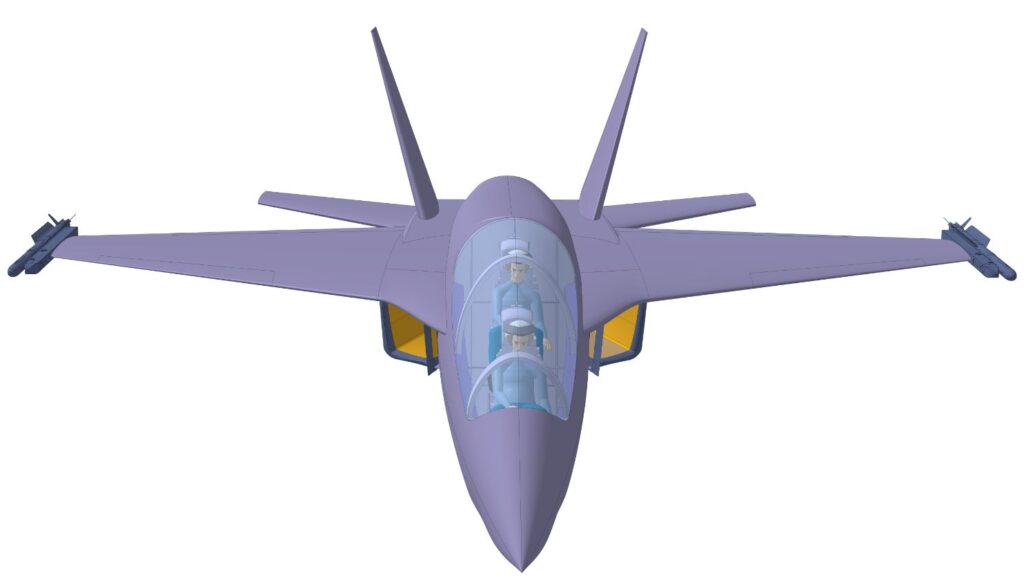
IAR NG – Front izometric view.
A Sophisticated Analytical Solution
The team’s analytical method begins by considering an elementary point within the elliptical contact area. At this point, there’s a normal force pressing the tyre against the runway and a friction force that resists motion. The elementary friction force at any point depends on both the normal force at that location and the coefficient of friction between the tyre and runway surface.
The steering friction moment is calculated by multiplying each elementary friction force by its distance from the centre of rotation. To find the total steering friction moment, the researchers integrated these elementary moments over the entire elliptical contact area. This integration process involves complex mathematical techniques. For practical calculations, the team converted this formula into a numerical approximation that can be easily implemented in engineering software like Mathcad.
Real-World Application for Aircraft Safety
Using data from the IAR 99NG military training aircraft, the research team applied this analytical method to calculate the steering friction moment. With a taxiing tyre deflection of 33 mm, contact area dimensions of 136.8 mm by 61.2 mm, and a friction coefficient of 0.31, the analytical method yielded a steering friction moment of 364.17 Newton-metres.
This value is crucial for designing the steering actuators. Aircraft designers typically dimension the active moment developed by the steering actuators to be at least 1.5 times greater than the calculated resisting moment. This safety margin ensures reliable steering performance even under adverse conditions.
From Theory to Practice
This innovative research bridges theoretical mathematics and practical aerospace engineering. The analytical method developed by the team provides a highly accurate way to calculate the steering friction moment, ensuring that military training aircraft can be safely steered during ground operations.
The researchers point out that their analytical method can be implemented in Mathcad, making it accessible to aerospace engineers working on aircraft design. This computational approach allows for precise calculations that would be tedious or impractical to perform by hand. The team also notes that they plan to validate their analytical method through experimental testing in the near future. This experimental validation will provide additional confidence in the mathematical models and further improve the safety of military training aircraft.
Future Research Directions
The research team is currently working within the NUCLEU project, supported by the Romanian Ministry of Education and Research, to further advance their work on aircraft ground handling dynamics. They aim to perform experimental validation that will compare actual measurements with their theoretical predictions.
This research exemplifies how sophisticated mathematical modelling contributes to aircraft safety. By precisely calculating the steering friction moment, engineers can ensure that pilots maintain control of their aircraft during the critical phases of taxiing, take-off, and landing. It also has implications beyond military training aircraft. The same mathematical principles could be applied to commercial aircraft, potentially improving the safety and reliability of ground operations across the aviation industry.
The Importance of Accurate Engineering
Aircraft design involves countless calculations, each critical to the safety and performance of the final product. The researchers’ work on steering friction moment may seem like a specialised detail, but it represents the meticulous approach required in aerospace engineering. For pilots of military training aircraft, these calculations translate into equipment they can trust. When they turn the control wheel to steer their aircraft on the runway, they rely on steering systems designed with these precise calculations in mind.
This innovative analytical method ensures that when pilots need to steer, their commands are reliably transmitted to the nose wheel, maintaining control even under challenging conditions. This reliability is essential not only for normal operations but also for emergency situations where precise control can make the difference between safety and disaster. The team’s research reminds us that behind every safe flight are countless hours of engineering, mathematics, and testing – all working together to keep aircraft and their crews secure both in the air and on the ground.
SHARE
DOWNLOAD E-BOOK
REFERENCE
https://doi.org/10.33548/SCIENTIA1271
MEET THE RESEARCHER
Dr Bogdan Adrian Nicolin
INCAS (National Institute for Aerospace Research), Bucharest, Romania
Dr Bogdan Adrian Nicolin is an Aerospace Researcher at the National Institute for Aerospace Research in Bucharest, Romania. He brings 15 years of professional experience, including over 8 years specialising in aircraft design, focusing on primary and secondary structures, life support systems, environmental control systems, and landing gear development. Dr Nicolin earned his Doctoral degree in Industrial Engineering and Robotics from the Polytechnic University of Bucharest (2018–2025), with research focused on improving the performance of nose landing gear for military training aircraft. He previously completed a Master’s degree in Quality in Engineering and Business Management (2012) and a Bachelor’s degree in Industrial Economics Engineering (2010), both from the Polytechnic University of Bucharest. His interdisciplinary background combines engineering expertise with business and quality management principles.
CONTACT
W: www.incas.ro
X: https://www.researchgate.net/profile/Nicolin-Adrian
https://www.webofscience.com/wos/author/record/ACS-0390-2022
https://orcid.org/0000-0002-5310-3981
https://scholar.google.com/citations?user=uzo6YIgAAAAJ&hl=ro
Dr Ilie Nicolin
INCAS (National Institute for Aerospace Research), Bucharest, Romania
Dr Ilie Nicolin is a Senior Aerospace Researcher and Head of the Technological and Conceptual Development Unit at the National Institute for Aerospace Research in Bucharest, Romania. With over 40 years of professional experience, including 36 years specialising in aircraft design and design verification, his expertise spans hydraulic systems, primary and secondary structures, flight controls, and landing gear development. Dr Nicolin obtained his Doctoral degree in Tribology and Machine Parts from the Polytechnic University of Bucharest in 1998, with research focused on sealing mechanisms for aircraft hydraulic systems. He earned his Engineering degree in Technology of Machine Design from the same institution in 1985. His extensive career combines deep technical knowledge with leadership in aerospace innovation and technological development.
CONTACT
W: www.incas.ro
X: https://www.researchgate.net/profile/Ilie-Nicolin
https://orcid.org/0000-0002-5920-3190
https://www.webofscience.com/wos/author/record/ADD-5119-2022
https://scholar.google.com/citations?user=W_AxkY0AAAAJ&hl=en
FUNDING
Romanian Ministry of Research, Innovation, and Digitisation
FURTHER READING
AB Nicolin, I Nicolin, Calculation of steering system parameters of a military training aircraft, Macromolecular Symposia, 2024, 413, 2300157. DOI: https://doi.org/10.1002/masy.202300157
REPUBLISH OUR ARTICLES
We encourage all formats of sharing and republishing of our articles. Whether you want to host on your website, publication or blog, we welcome this. Find out more
Creative Commons Licence (CC BY 4.0)
This work is licensed under a Creative Commons Attribution 4.0 International License.
What does this mean?
Share: You can copy and redistribute the material in any medium or format
Adapt: You can change, and build upon the material for any purpose, even commercially.
Credit: You must give appropriate credit, provide a link to the license, and indicate if changes were made.
SUBSCRIBE NOW
Follow Us
MORE ARTICLES YOU MAY LIKE
Dr Sang-Joon Cho | 3D Atomic Force Microscopy: Overcoming Challenges in Nano-Scale Measurement
Nano-scale imaging and measurement are crucial for the development of new gadgets – from the latest phones to advanced drug discovery technologies. Dr Sang-Joon Cho and his team at Park Systems Corporation have developed a new approach to measuring and characterising microscopic components, offering exciting potential to accelerate advancements in the technologies essential to the modern world.
Advanced Air Mobility National Campaign: Bridging the Gap
The rapid advancements in technology have paved the way for revolutionary changes in transportation, particularly air mobility. One such groundbreaking initiative is the Advanced Air Mobility (AAM) National Campaign led by NASA. This campaign aims to integrate advanced air mobility solutions into the existing transportation infrastructure, creating a seamless, efficient, and safe urban air transportation system. By addressing the various challenges associated with urban air mobility, the AAM National Campaign is poised to redefine how we navigate our cities, ultimately leading to reduced congestion, improved accessibility, and enhanced environmental sustainability.
Dr Niloofar Vardian | Mapping the Unknown: Inside Black Holes
Dr Niloofar Vardian at the SISSA school has advanced our understanding of black hole interiors through precise mathematical modelling. Her recent publication sheds light on previously inaccessible aspects of black hole dynamics, deepening our knowledge of these mysterious and difficult-to-study phenomena.
Professor Edward Smith | Soaring to New Heights: Investigating the Aeroelastic Stability of Composite Aircraft Wings
Combining the best aspects of helicopters and fixed-wing aircraft into a single vehicle has been a long-held ambition in the aerospace industry. From Leonardo da Vinci’s early drawings of an ‘aerial screw’ to the V-22 Osprey tiltrotor aircraft used by the U.S. military today, engineers have grappled with the challenge of creating aircraft capable of both vertical take-off and landing (VTOL) and high-speed forward flight. While helicopters offer unparalleled VTOL performance, their top speeds are limited to around 300 km/h. Fixed-wing aircraft offer greater speed but require long runways for take-off and landing. Reconciling these conflicting design requirements is a major challenge.