Changing the Brain Through Learning
Attention allows us to plan and monitor our thoughts and, thus, is a critical step in the learning process. Learning can then change the physical structure of the brain. This is the reason why a team of scientists at the University of Oregon, led by Drs Michael Posner and Cristopher Niell, are exploring the effects of attention on learning and how learning changes the brain.
Learning: What, Why, How?
To learn means to acquire specific skills, knowledge and understanding through study or experience. In humans, scientists can measure the quality and success of our learning by looking at different variables like speed or the number of errors on tests specifically designed to measure this aspect of our cognitive function. Typically, learning can be determined through a reduction in the number of errors made and/or completing a task more quickly.
This experimental approach allows scientists to closely examine the factors that can contribute to learning. These factors include effort, prior experience and learning strategies. However, when we think about learning at the mechanistic level in the brain, the involvement of different brain regions in the cascade of the learning process is the primary focus. Dr Michael Posner of the University of Oregon has dedicated his career to advancing our understanding of how attention is organised in the human brain and its vitally important functions. The work we discuss here is the fruit of a collaborative effort between Dr Posner and his esteemed colleagues, all of whom are working towards the common goal of advancing cognitive neuroscience.
In a study published in 2019, Dr Aldis Weible (the lead author) and colleagues explored the mechanisms of learning in mice. The team focused on three critical brain regions – the anterior cingulate cortex, hippocampus and visual cortex. The anterior cingulate cortex is involved in attentional control. The primary role of the hippocampus is memory and learning regulation. Both structures are located deep in the brain’s subcortical regions. The visual cortex receives and processes visual information from our eyes and processes this with the aid of attention and other brain areas. The process of learning involves all three of these brain areas (and others) that, together, form a very complex network.
This study filled some key gaps in our understanding of learning. The team discovered that each region contributes to the complex network of learning. However, they also found that this contribution fits better with a parallel rather than a serial model. To explain this, they used a racehorse analogy, whereby the brain regions work together at the same time. The anterior cingulate cortex and the visual cortex were found to be important at all stages of learning, while the hippocampus influenced mainly the middle and late stages. However, there were also differences in how these brain regions were involved. For example, the anterior cingulate cortex was linked to accuracy performance but not speed.
These findings were surprising because many previous studies had linked attention to reduced learning speed. From this novel result, it was clear that more answers could be uncovered by exploring further the connections between the anterior cingulate cortex and other brain areas.
Bringing All the Evidence Together
In one of his most recent pieces of work, Dr Cristopher Niell and colleagues collaborated with Drs Posner and Rothbart to review the scientific evidence on learning. Their combined expertise spanning neuroscience, psychology and biology allowed them to ask questions about similarities and differences between animals and humans when it comes to learning new skills. They already knew what brain regions appeared to be important in the learning process, but there was a lot more they needed to unravel about the role of attention and memory networks. Are there any special connections involved? Do they look similar in mice and in humans? And intriguingly, if we find out more about these pathways and the mechanisms behind them, could we manipulate them to improve learning?
The team was able to provide answers to several of these questions. Notably, they found that the evidence pointed towards the presence of two pathways in the brains of both animals and humans, serving to connect attentional networks with the hippocampus. The first pathway (anterior pathway) involves the brain structure nearer the front of the brain and includes the anterior cingulate cortex. The second pathway (posterior pathway) involves the brain structures further back, including areas of the parietal lobe.
Because these pathways use different routes crossing many brain structures, the team speculated about the mechanisms that might underpin learning. Most specifically, they were interested in whether the anterior pathway could be significant for generalisation – a process that helps the brain demonstrate learning in situations different from the original learning. They further proposed that the posterior pathway might be complementary to this and may play a part in navigation in the rodent and retrieval of memories related to location in the human.
Manipulating Pathways Through Neuromodulation
Neuromodulation is a technique through which we can actively change the brain’s nerve activity using mild electrical stimulation or drugs. Based on encouraging results from the field of epilepsy, where neuromodulation can reduce seizures, researchers were encouraged to study its potential application to other disorders, as well as the understanding of healthy brain processes.
For Dr Posner, neuromodulation presented a very promising candidate for improving learning outcomes. Based on his earlier research, he was eager to explore the anterior cingulate cortex and surrounding brain structures, particularly in relation to improving learning. To look at this directly, the team used lasers to manipulate neuronal activity in mice in the anterior cingulate cortex.
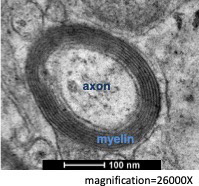
An electron microscope picture of a single axon (fibre that leads to another cell) surrounded by myelin rings (insulating the axon).Electron microscopy by Dr Denise Piscopo
Neuromodulation Can Repair the Brain
Neuromodulation of the anterior cingulate cortex of mice by low-frequency stimulation resulted in several exciting results. First, they showed that the brain structure close to the stimulation became more strongly connected after neuromodulation. White matter is the tissue that allows communication from one part of the brain to another. Dr Denise Piscopo (lead author), was able to take pictures magnified 16,000 times to show an increase in the white matter surrounding the anterior cingulate, allowing information to travel more quickly and more effectively to and from this structure (see Figure 2).
This was excellent news – not least because neuromodulation might help to repair the protective sheet of white matter across many different neurological problems. For example, people who suffer from concussions or multiple sclerosis have damage to white matter in diverse brain regions. These findings suggest a possible way forward to help the recovery of patients with such neurological problems.
Neuromodulation and Meditation
Plenty of evidence supports the positive effects of meditation on the brain. Some work by Drs Posner and Rothbart in conjunction with Dr Yiyuan Tang has shown that brain connections strengthened after two to four weeks of meditation training. Could meditation have similar positive effects on the brain as neuromodulation? They identified several common patterns in the literature pointing to the role of the anterior cingulate cortex, stronger white matter, and better learning outcomes on different tests and tasks using meditation training in humans and stimulation in animals.
Neuromodulation and meditation seem to involve a specific brain rhythm that causes a cascade of molecular changes in the brain. Dr Pascale Voelker is investigating these molecular changes. Taken together, both neuromodulation and mental activity, such as meditation, are believed to improve information transportation by strengthening white matter connections. This impacts real-life behavioural outcomes, such as improving concentration and emotional regulation. But how?
Next Steps in Understanding Learning
Having ascertained that neuromodulation can improve connections between structures, it is important to understand the mechanisms underlying the impact neuromodulation has on the two pathways involved in learning skills. One question is how to separate the direction of information flow in the two pathways. Understanding the direction of information flow will help determine the exact function of each pathway between attention and memory. Neuromodulation could then be targeted to help understand the function of each pathway. Hopefully, modulation can then be used to improve specific learning skills. In the long run, improved knowledge could repair white matter and thus help people to recover from neurological problems.
But, of course, very little is ever straightforward. As Dr Posner explains, ‘The ability to determine the direction of information flow is critical to the design of new studies. It is hard to distinguish between memory-to-attention and attention-to-memory control in subcortical areas. Optogenetics using laser light can help them to accomplish some of these goals. By injecting the brains of mice with two different types of viruses, they will be able to separate the direction of two pathways. It is then possible to use laser light to stimulate these areas in a specific direction through surgically implanted optic fibres.
Future research could involve designing tasks for mice that would help in modulating each pathway separately. This experimental plan is similar to the researchers’ previous studies looking at the contribution of different brain areas to learning. This will allow study of the specific roles these pathways have and how the information flows within them. Looking even further ahead, if successful, it may then be possible to apply similar non-invasive methods, such as stimulation from electrodes on the scalp of humans, to improve both neurological conditions and skill learning.
SHARE
DOWNLOAD E-BOOK
REFERENCE
https://doi.org/10.33548/SCIENTIA923
MEET THE RESEARCHER
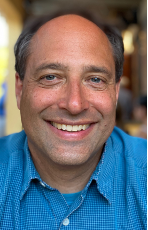
Professor Cristopher Niell
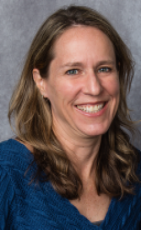
Dr Denise Piscopo
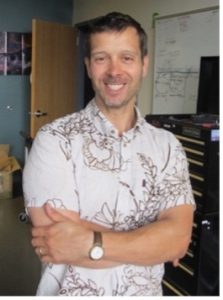
Dr Aldis P. Weible
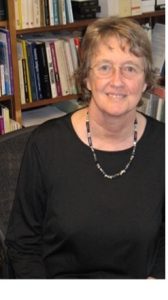
Professor Mary K. Rothbart
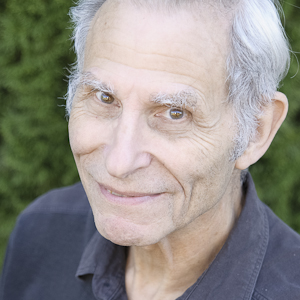
Professor Michael Posner
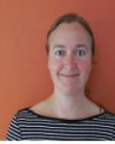
Dr Pascale Voelker
Team Profile
Departments of Psychology & Biology and the Institute of Neuroscience
University of Oregon
Eugene, OR
USA
This team began its collaboration in 2015 when after a lecture by Dr Posner, Dr Niell came forward to say that he could provide a test of Dr Posner’s hypothesis that meditation could change through a frontal rhythm that might activate dormant cells. Dr Niell was using optogenetics to activate cells in the brain of mice. In addition to Dr Posner, who has studied the mechanisms of attention for more than 50 years, the team included Dr Mary K. Rothbart, a world-leading developmental psychologist with whom Dr Posner has worked for many years. Dr Denise Piscopo (working with Dr Niell) was instrumental in testing the hypothesis and developed the needed skill with electron microscopes to examine the change in white matter. Dr Aldis Weible worked with Dr Niell to train and test the mice, for which he received special recognition with a research award from the University. Dr Pacale Voelker, trained in molecular biology, added the molecular analysis to the team’s work. The experiments reported in this article reflect a genuine collaboration and also build on the work of many other people in this field.
CONTACT
W: https://psychology.uoregon.edu/profile/mposner/
KEY COLLABORATORS
Dr Cristopher Niell, University of Oregon
Dr Pascale Voelker, University of Oregon
Dr Denise Niell, University of Oregon
Dr Mary Rothbart, University of Oregon
Dr Aldis Wieble, University of Oregon
Dr Gary Lynch, University of California Irvine
Dr Yiyuan Tang, Arizona State University
FUNDING
This work was supported by grants from the Office of Naval Research to the University of Oregon. The team wishes to express its appreciation to the late Dr Ray Perez, who approached us with the goal of using our research to develop a model of skill acquisition. We also acknowledge the contribution of Dr Harold Hawkins, ONR, and Mr Rudy Chapa, who both contributed funding to our research.
FURTHER READING
MI Posner, AP Weible, P Voelker, et al., Decision Making as a Learned Skill in Mice and Humans, Frontiers in Neuroscience, 2022, 16. DOI: https://doi.org/10.3389/fnins.2022.834701
AP Weible, MI Posner, CM Niell, Differential Involvement of Three Brain Regions During Mouse Skill Learning, eNeuro, 2019, 6(4). DOI: https://doi.org/10.1523/ENEURO.0143-19.2019
DM Piscopo, AP Weible, MK Rothbart, et al., Changes in white matter in mice resulting from low-frequency brain stimulation, Proceedings of the National Academy of Sciences of the United States of America, 2018, 115(27), E6339–E6346. DOI: https://doi.org/10.1073/pnas.1802160115
MI Posner, YY Tang, G Lynch, Mechanisms of white matter change induced by meditation training, Frontiers in Psychology, 2014, 5, 1220. DOI: https://doi.org/10.3389/fpsyg.2014.01220
REPUBLISH OUR ARTICLES
We encourage all formats of sharing and republishing of our articles. Whether you want to host on your website, publication or blog, we welcome this. Find out more
Creative Commons Licence (CC BY 4.0)
This work is licensed under a Creative Commons Attribution 4.0 International License.
What does this mean?
Share: You can copy and redistribute the material in any medium or format
Adapt: You can change, and build upon the material for any purpose, even commercially.
Credit: You must give appropriate credit, provide a link to the license, and indicate if changes were made.
SUBSCRIBE NOW
Follow Us
MORE ARTICLES YOU MAY LIKE
Dr Michael Hicks | Creating Skeletal Muscle from Stem Cells
Recognising the precise steps involved in the differentiation process of stem cells into various cell types is crucial to regenerative medicine. Researchers face the specific challenge of how to fine-tune and optimise protocols for directing stem cells to differentiate into specific cell lineages. As such, the directed differentiation to skeletal muscles has not yet advanced to the clinical trials stage. Dr. Michael Hicks from the School of Medicine at the University of California, Irvine, is working to change this with his extensive studies into the transition of stem cells into skeletal muscles.
Dr Tomonori Kaifu | Battling Bone Diseases: The Intriguing Roles of the Dendritic Cell Immunoreceptor
Unravelling the complex communication pathways between cells is vital to identifying new therapeutic targets for certain bone diseases. Dr Tomonori Kaifu is based at the Tohoku Medical and Pharmaceutical University in Japan. Over the years, his research has focused on cell signalling via the dendritic cell immunoreceptor. He works to understand the various roles of this receptor in regulating the immune system and metabolism of the bones, with the aim of developing novel therapies for metabolic bone diseases and autoimmune conditions.
Dr Dagmar Zeithamova | How Our Brains Create Categories: A Look Inside the Mind
Our brains have an incredible ability to organise information into meaningful categories, allowing us to make sense of the world around us. However, this categorisation process can also bias how we perceive and remember this world. Dr Dagmar Zeithamova and her team at the University of Oregon are uncovering how our brains form category-biased representations, even when we’re focused on learning about individual examples. Their work sheds new light on the neural mechanisms underlying concept formation and how categories shape our perceptions.
Improving Joint Replacement Materials
Hip and knee replacements are key for the health and well-being of many people. Unfortunately, the materials used in these procedures are known to wear and corrode once placed within the human body. Dr Stefano Mischler and his team from École Polytechnique Fédérale de Lausanne, together with Professor Dr Brigitte Jolles-Haeberli and her team from Lausanne University Hospital in Switzerland, have conducted exciting new research that advances our understanding of the factors influencing corrosion in the human body. Their work has exciting implications for the future monitoring of implant conditions, particularly valuable in the face of our increasingly ageing population.