Dr Arpita Bose – Harnessing Microbes to Produce Sustainable Plastics and Biofuels
Before oxygen was widely available in Earth’s atmosphere, ancient microbes looked to other elements to obtain electrons for photosynthesis. Some of these microbes are called ‘photoferroautotrophs’ – which can take up electrons from iron available in their surrounding environment and use them to transform carbon dioxide (CO2) into biomolecules. In their research, Dr Arpita Bose and her team at Washington University in St Louis, explore the mechanisms these microbes exploit to produce biomolecules, using the electrons they take in. Their discoveries are leading to sustainable new ways to produce both plastic and fuel – and could soon prove to reduce our reliance on the compounds derived from crude oil.
Microbial Electrosynthesis
Microorganisms have evolved a bewildering array of techniques to obtain nutrients from their surrounding environments. As biologists learn more about these methods, their potential use in our technology is now looking increasingly promising. Perhaps one of the most fascinating of these feeding methods is called ‘microbial electrosynthesis’.
The process can be controlled using devices named ‘microbial electrochemical cells’ powered by electricity, in which oppositely-charged electrodes are placed in a charge-carrying electrolyte. By attaching themselves to the device’s negatively-charged cathode, photoautotrophic microbes that feed through microbial electrosynthesis can use the abundant incoming electrons to catalyse biochemical photosynthesis reactions, and convert CO2 into value-added multi-carbon compounds.
By exploiting these behaviours, Dr Arpita Bose and her team at Washington University hope that they can mitigate some of humanity’s most environmentally-damaging activities, including plastic use. However, many improvements are needed before these techniques can be rolled out on industrial scales. In their research, Dr Bose and her team explore this issue in detail, drawing from the latest advances in chemistry, microbiology, and materials science.
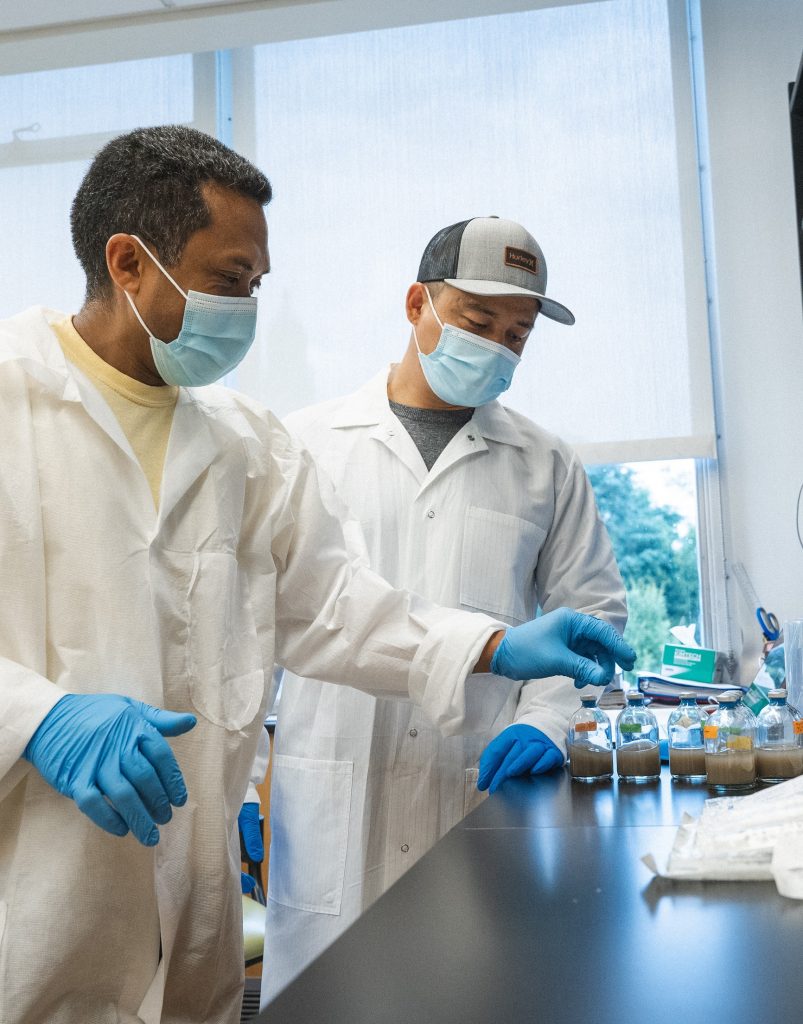
CREDIT: Sean Garcia, Washington
University in St. Louis
Enhancing Electron Uptake
In previous studies by the Bose team, promising opportunities for improving the performance of microbial electrosynthesis emerged with the use of a rod-shaped photosynthetic bacterium named Rhodopseudomonas palustris TIE-1 (TIE-1) – which takes up electrons using a surface protein system called Pio. In principle, this mechanism can be exploited to manufacture useful biomolecules sustainably – but before applying it in real-world applications, researchers would need to find ways to improve the growth and electron uptake ability of TIE-1.
In a 2018 study, Dr Bose and her team took an important step towards this goal by examining the interaction of TIE-1 with various forms of iron as a source of electrons – a behaviour known as ‘photoferroautotrophy’. This microbial metabolism is widely believed by biologists to be one of the most ancient forms of photosynthesis.
In their experiment, the team explored how microbial electrosynthesis in TIE-1 could be enhanced with the inclusion of different forms of iron. They discovered that while electron uptake didn’t noticeably change when iron was dissolved in the electrolyte, it improved significantly when an insoluble iron-based compound named Prussian blue was deposited as a film onto the electrochemical cell’s graphite cathode. With this simple addition, TIE-1 took in 4.2 times as many electrons as the uncoated cathode, paving the way for their more widespread application in the synthesis of value-added biochemicals.
Exploring Cytochromes
Despite this knowledge, the mechanisms used by TIE-1 to take in electrons were still poorly understood. Leading on from their discovery, Dr Bose and her team wished to explore the process in more detail. In their next study, published the following year in 2019, the researchers shed new light on this uptake process – again using Rhodopseudomonas palustris TIE-1 as a model. Through their analysis, they found that photoferroautotrophy in TIE-1 originates from specialised proteins named ‘cytochromes’, which harbour iron-containing molecules called ‘hemes’ at their core.
More specifically, this metabolism is driven by a system containing a cytochrome (PioA) and a porin (PioB), found in the bacterial periplasm and outer membrane, respectively. Together, this two-protein complex enables photoferroautotrophic microbes such as TIE-1 to transfer electrons from iron-based donors to biomolecular acceptors. TIE-1 can take up electrons from a number of possible electron sources, such as soluble iron or certain insoluble minerals and poised electrodes.
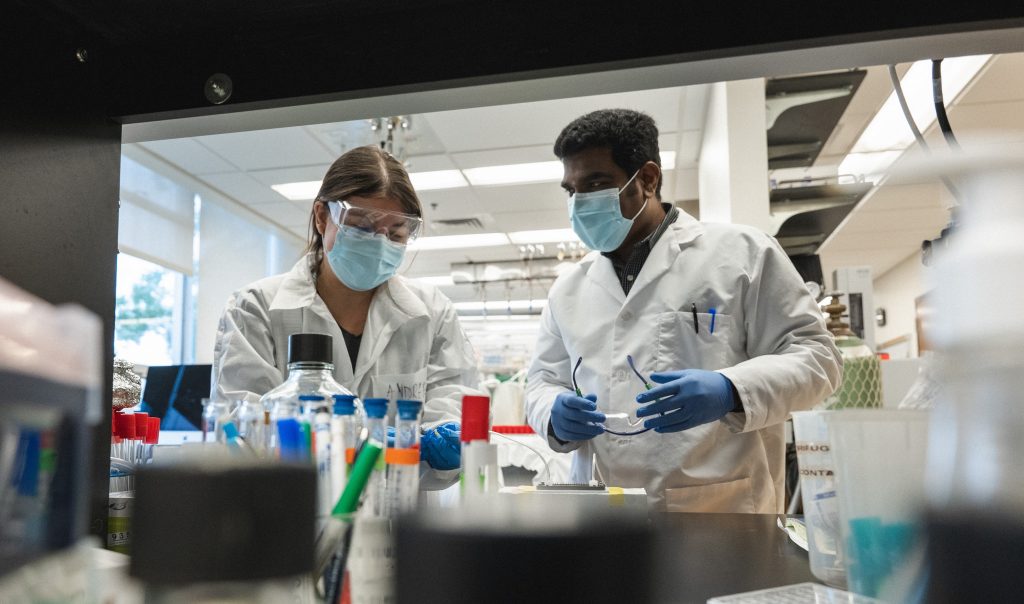
CREDIT: Sean Garcia, Washington University in St. Louis
Captured Electrons Used for Carbon Fixation
Alongside this important discovery, one additional aspect of the electron uptake process needed additional clarification – this time, concerning the link between electron uptake and CO2 fixation within the microbes. In this crucial stage of the microbial electrosynthesis process, the carbon contained in CO2 is converted into larger, more complex organic molecules, which are used as precursors for microbial metabolism and growth. However, after cytochrome-initiated electron capture by TIE-1, the mechanisms by which electrons are transferred to CO2, triggering the fixation process, were still poorly understood.
In a further 2019 study, Dr Bose and her team again used Rhodopseudomonas palustris TIE-1 contained in a microbial electrochemical cell to show how this electron transport is strongly correlated with the highly reducing, or electron-depleting nature of redox reactions within the microbial cell. They discovered that the process is driven by a particular CO2-fixing enzyme named ruBisCO. This enzyme is part of the well-studied ‘Calvin-Benson-Bassham’ cycle. This pathway allows the fixation of CO2 into more useful organic compounds.
The researchers clearly proved the importance of the process by deleting the genes that encode the ruBisCO enzyme – reducing TIE-1’s ability to take up electrons by as much as 90%. With this discovery, Dr Bose and her team now had a far more complete picture of how photoferroautotrophic microbes use their surrounding environments to survive.
Manufacturing Bioplastics
Having studied these biomechanisms in a suitable level of detail, the team could now turn their attention to exploring their potential real-world applications. Among these is the production of bioplastics named ‘polyhydroxybutyrates’ (PHBs). This family of biodegradable polyesters can be produced naturally inside several different types of bacteria. In an additional 2019 study, Dr Bose and her team assessed the ability of Rhodopseudomonas palustris TIE-1 to produce PHBs under various conditions including the use of a microbial electrochemical cell.
In the experiments, various tests were performed, to obtain PHB as a product of CO2 fixation. The researchers found that although TIE-1’s ability to produce PHB varied in different conditions, electron capture (from iron or poised electrodes) through photoferroautotrophy was among the most effective processes available for PHB production. Through further studies of TIE-1’s gene expression, they suggest a future possibility to fine-tune and enhance PHB production by this microbe. These discoveries paved the way for additional studies of how sustainable bioplastics could be produced using widely available resources, including light, electricity, iron, and CO2.
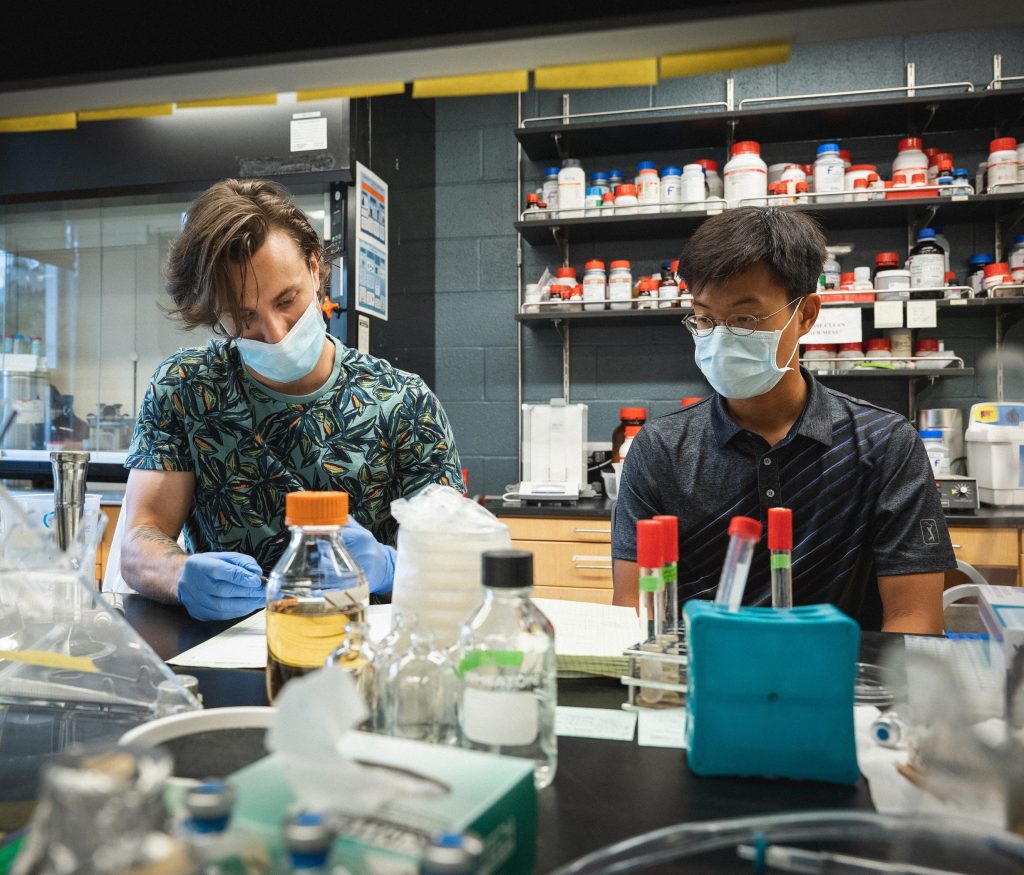
CREDIT: Sean Garcia, Washington University in St. Louis
Enhancing PHB Production
Before bioplastics can be manufactured on industrial scales, researchers will still need to address limitations in electron uptake in the specific context of PHB production within microbial electrochemical cells. In their latest study from 2020, Dr Bose and her team explored potential improvements to electron uptake, through modifications to the cell’s cathode. In their design, conductive materials were developed from a combination of nanoparticles, made from an iron oxide mineral named ‘magnetite’, and a supporting material named ‘graphene’, which is known for its highly advanced electrical properties.
After depositing these materials onto a carbon felt cathode, the researchers tested the performance in microbial electrochemical cells. Their experiments showed that TIE-1 produces around 4.2 times more PHB than an electrochemical cell with an unmodified carbon felt electrode; and up to 20 times as much as the yields achieved in previous studies, using standard graphite electrodes. The success of the experiment now presents clear routes to the industrial-scale production of sustainable PHB. This creates a pathway for sustainable bioplastic production that bypasses the need for the crude oil required for conventional plastic manufacturing.
Producing Sustainable Biofuels
Alongside their use in plastic production, organic molecules are also highly desired for the energy they release when combusted. In previous studies, researchers have explored the use of microbes such as cyanobacteria to produce sustainable biofuels. However, these organisms produce oxygen during photosynthesis. Biofuel synthesis can have limited efficiency when pathways involve oxygen-sensitive enzymes.
In a further 2020 study, Dr Bose and her team studied how TIE-1 could be exploited to produce a biofuel named n-butanol. As a fuel, n-butanol is particularly desirable for its high energy content and low tendency to vaporise or dissolve in water without combusting, compared with ethanol, a commonly used biofuel.
During their experiments, Dr Bose and her team constructed a mutant form of TIE-1 that could not fix nitrogen. This mutant is unable to grow when nitrogen gas is the only nitrogen source. As a result, more of the electrons captured by TIE-1 went towards the specific production of n-butanol – increasing the electron yield of the biofuel without a need to increase the cell’s electricity consumption significantly.
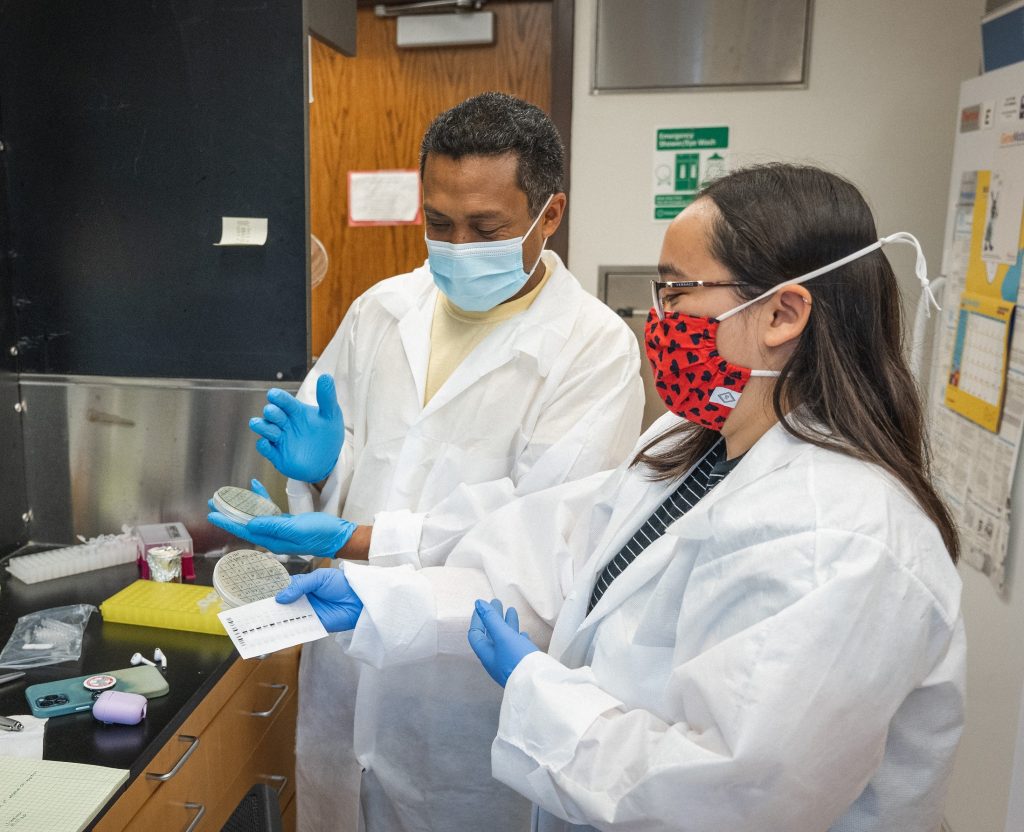
CREDIT: Sean Garcia, Washington University in St. Louis
Moving Away from Fossil Fuels
Today, even as the call to end our reliance on fossil fuels and unsustainable plastics grow increasingly urgent, humanity’s use of these hydrocarbons continues to grow. Through their studies, Dr Bose and her team have now shown how microbial electrosynthesis could be exploited to address these issues – producing both sustainable biofuels and bioplastics through TIE-1-driven CO2 fixation.
Alongside its sustainability, the widespread use of biodegradable PHB is highly desired in medical applications – with a biocompatibility that makes it suitable for procedures including drug delivery and reconstructive surgery. Industrial-scale manufacturing of bioplastics and biofuels using microbial electrosynthesis can be achieved using the electricity produced by solar panels – creating a fully sustainable cycle.
Ultimately, by exploiting a microbial metabolism that evolved in the distant past, Dr Bose and her team now hope that new methods will emerge that can address some of the most pressing problems of our time.
Reference
https://doi.org/10.33548/SCIENTIA730
Meet the researchers
Arpita Bose
Department of Biology
Washington University in Saint Louis
St Louis, MO
Dr Arpita Bose studies the mechanisms that microbes use to survive, and how they can be exploited to address issues related to energy production, pollution, and sustainability. After completing her PhD at the University of Illinois at Urbana, she worked as a research associate at institutions including MIT and Harvard University, before founding her own lab at Washington University in Saint Louis in 2015. In her world-leading research, Dr Bose aims to engineer microbial systems for the sustainable production biochemicals and bioenergy, and to tackle issues including the treatment of contaminated water and soil. She also aims to learn more about how microbial phenomena shape our planet, and possibly others.
E: abose@wustl.edu
W: https://biology.wustl.edu/people/arpita-bose
Tahina Ranaivoarisoa
Department of Biology
Washington University in Saint Louis
St Louis, MO
Tahina Ranaivoarisoa joined the laboratory of Dr Bose in 2015 and is now the laboratory manager. Prior to the role, he was a Laboratory Supervisor at the Ambatovy mining project in Madagascar, which he joined after completing his Masters’ degree in Biochemistry and Biotechnology at the University of Missouri St Louis in 2009. His roles in Dr Bose’s lab include designing and directing experiments involving Rhodopseudomonas palustris TIE-1 to produce bioplastics and biofuels. Alongside these roles, Ranaivoarisoa is responsible for training students on laboratory techniques.
E: ranaivoarisoao@wustl.edu
W: https://biology.wustl.edu/people/tahina-ranaivoarisoa
Rajesh Singh
Department of Biology
Washington University in Saint Louis
St Louis, MO
Dr Rajesh Singh joined the laboratory of Dr Bose in 2016 as a postdoctoral research associate and is now a staff scientist. His research centres around investigating microbial diversity in aquatic and terrestrial ecosystems, and studying how these microbes shape the ecology and environment. Dr Singh received his PhD from Miami University, Oxford, Ohio where he studied mineral/metal-microbe interactions, highlighting the potential implications of microbes in metal bioremediation. In the Bose lab, he is responsible for designing and conducting geomicrobiological experiments using freshwater and marine phototrophs to bring new insights on their role in the biogeochemical cycling of elements such as iron and carbon.
E: rsingh24@wustl.edu
W: https://biology.wustl.edu/people/rajesh-singh
Karthikeyan Rengasamy
Department of Biology
Washington University in Saint Louis
St Louis, MO
Dr Karthikeyan Rengasamy is a staff scientist in the Bose lab. He received his PhD in microbial electrochemical technology from the Central Electrochemical Research Institute in India. He has acquired experience in the characterisation of electroactive biofilms using various electrochemical analytical techniques to understand microbial electron transfer. Currently, Dr Rengasamy is working on various novel aspects of photosensitive microbes with charged electrodes. His research focuses on understanding electromicrobiology under different environments including light, dark, fresh water, sea water and various wetland soils.
E: karthikeyanrengasamy@wustl.edu
Wei Bai
Amyris Inc
Emeryville, CA
Dr Wei Bai joined the Bose lab in 2015 as a research assistant. She received her PhD in Energy, Environment & Chemical engineering from Washington University in St. Louis in 2020. Her research in the Bose lab focused on investigating the possibility of using sustainable and naturally abundant resources to synthesise carbon-neutral biofuels with a purple non-sulfur bacterium. She also developed novel genetic engineering tools with high efficiency and accuracy. Currently, Dr Bai is a scientist at Amyris. Inc, working on designing and constructing genetically modified yeast for target molecule production from sustainable resources using metabolic engineering techniques.
E: bai@amyris.com
FUNDING
This work was supported by the following grants to AB: The David and Lucile Packard Foundation Fellowship (201563111), the US Department of Energy (grant number DESC0014613), and the US Department of Defense, Army Research Office (grant number W911NF-18-1-0037), Gordon and Betty Moore Foundation, National Science Foundation (Grant Numbers 2021822 and 2124088), Air Force Office of Scientific Research DEPSCoR (Grant Number FA95502110211) and the US Department of Energy by Lawrence Livermore National Laboratory under Contract DEAC5207NA27344 (LLNL-JRNL-812309). AB was also funded by a Collaboration Initiation Grant, an Office of the Vice-Chancellor of Research Grant, and an International Center for Energy, Environment, and Sustainability Grant from Washington University in St. Louis. Students are also supported by an Institutional Training Grant in Genomic Science from the NIH (T32 HG000045-18) and the Initiative for Maximizing Student Development (IMSD) training grant from the US National Institutes of Health (grant number R25-GM103757).
Want to republish our articles?
We encourage all formats of sharing and republishing of our articles. Whether you want to host on your website, publication or blog, we welcome this. Find out more
Creative Commons Licence
(CC BY 4.0)
This work is licensed under a Creative Commons Attribution 4.0 International License.
What does this mean?
Share: You can copy and redistribute the material in any medium or format
Adapt: You can change, and build upon the material for any purpose, even commercially.
Credit: You must give appropriate credit, provide a link to the license, and indicate if changes were made.
More articles you may like
Dr Lifei Wang | Can Species Distribution Models Inform Us About Future Ecosystems?
The world is buzzing with news about how human activities and climate shifts are reshaping our ecosystems. Have you ever wondered how life will adapt to this rapidly changing world? Ecologists might be able to predict how different species will live in future using computer simulations. Dr Lifei Wang at the University of Toronto Scarborough investigates how different stimulations work under varying conditions to provide new insights into what may lie ahead.
Dr Yong Teng | Improving the Outlook for Head and Neck Cancer Patients
Dr Yong Teng at the Emory University School of Medicine is working with colleagues to overcome the high mortality of individuals diagnosed with cancers affecting the head and neck. One of his approaches is based on understanding the particular mechanisms of the ATAD3A gene, which new insights suggest are closely related to cancers affecting the head and neck.
Dr Tsun-Kong Sham – Dr Jiatang Chen – Dr Zou Finfrock – Dr Zhiqiang Wang | X-Rays Shine Light on Fuel Cell Catalysts
Understanding the electronic behaviour of fuel cell catalysts can be difficult using standard experimental techniques, although this knowledge is critical to their fine-tuning and optimisation. Dr Jiatang Chen at the University of Western Ontario works with colleagues to use the cutting-edge valence-to-core X-ray emission spectroscopy method to determine the precise electronic effects of altering the amounts of platinum and nickel in platinum-nickel catalysts used in fuel cells. Their research demonstrates the potential application of this technique to analysing battery materials, catalysts, and even cancer drug molecules.
Dr Michael Cherney – Professor Daniel Fisher | Unlocking Woolly Mammoth Mysteries: Tusks as Hormone Time Capsules
The impressive tusks found on proboscideans (the order of mammals that includes elephants, woolly mammoths, and mastodons) are like time capsules, preserving detailed records of their bearers’ lives in the form of growth layers and chemical traces. Frozen in time for thousands of years, these layers can unlock secrets about the lives of long-extinct relatives of modern elephants. Dr Michael Cherney and Professor Daniel Fisher from the University of Michigan used innovative techniques to extract and analyse steroid hormones preserved in woolly mammoth tusks. This ground-breaking work opens new avenues for exploring the biology and behaviour of extinct species.