Dr Badih Assaf | Dr Xinyu Liu – Building Topological Superconductors for Quantum Computers
Despite the numerous opportunities presented by quantum computers, their practical use has so far been hindered by their vulnerability to the surrounding environments. Dr Badih Assaf, Dr Xinyu Liu and their colleagues at the University of Notre Dame, Indiana, show how these problems could be overcome, through the use of an exotic class of hybridised materials named ‘topological superconductors’. Through the optimised fabrication of these materials, and a detailed analysis of their quantum properties, the team’s results could pave the way for the widespread use of topological superconductors in robust and practical quantum computers.
Quantum Computing
Today, quantum computing is among the fastest growing and most widely studied fields of technology. By harnessing the often-enigmatic properties of quantum superposition, interference, and entanglement, these devices allow information to be stored on quantum bits, or ‘qubits’. Currently, qubits can come in the form of the spins of single electrons, or the polarisations of individual particles of light, called photons.
Crucially, whereas a conventional computer bit can only occupy one of two states – 0 or 1 – a qubit can exist in a superposition of both states simultaneously. In addition, where the amount of information stored by a conventional computer can only scale linearly with their numbers of bits, the number of bits stored by quantum computer can be described as 2 to the power of the number of entangled qubits it contains. That means that theoretically, a system containing just dozens of qubits could be used to solve problems vastly more complex than the most advanced supercomputers available today.
However, the technology faces a number of complex challenges before we can begin to see its widespread use in everyday scenarios. ‘One of the key issues with quantum computing devices is their sensitivity to the environment,’ explains Dr Badih Assaf of the University of Notre Dame.
Systems of entangled qubits are incredibly delicate. If they are able to interact with the outside world, even the subtlest vibrations generated by heat can cause them to lose the quantum information they carry. Preventing this interaction currently requires exceptionally intricate and expensive equipment – but through their research, Dr Assaf and his colleague Dr Liu explore a newly-emerging, more advantageous solution to this immense challenge.
Topological Superconductors
In conventional computers, information is often stored using materials named semiconductors, whose electrical conductivities fall in between those of conductors and insulators. In modern computers, semiconductors are used to construct logic gates, whose conductivity can be controlled by varying the voltage across an external gate terminal – allowing operators to switch states between 0 and 1.
Since this innovation became widespread, new materials have been discovered that host more advanced electrical properties. Among these are superconductors, which display zero resistance to electrical current when cooled to ultra-low temperatures. Even more exotic characteristics can be found in materials named ‘topological insulators’ – whose outer surfaces act as robust electrical conductors, but whose interiors behave like insulators, making it far harder for electrons to move around.
If superconductors and topological insulators are combined into a single, hybrid device, a structure emerges that exhibits the advantageous properties of both types of material. ‘This specific type of superconductor is dubbed the topological superconductor, and it is rare in nature,’ Dr Assaf describes. ‘It enables the generation of new quantum effects that are robustly protected from the environment.’ As a result, Dr Assaf and Dr Liu believe that topological superconductors hold much promise in overcoming the challenges posed by lost quantum information in existing quantum computers.
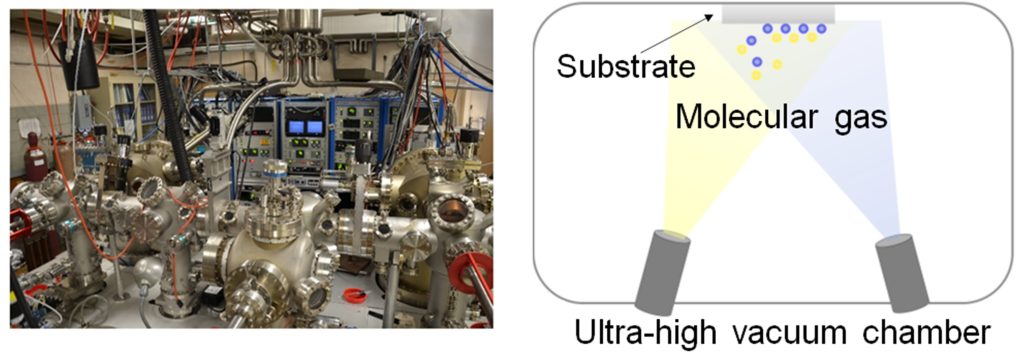
Figure 1 (left) Notre Dame Molecular Beam Epitaxy cluster; (right) Sketch of the MBE deposition process.
Exotic Quasiparticles
The unique characteristics of topological superconductors are thought to arise from a certain type of ‘quasiparticle’, which emerges at the interface between a superconductor and a topological insulator. Quasiparticles are often found within solid materials, and behave much like individual particles – but are actually the result of more complex systems of particles, and their interactions with each other. They are not fundamental particles, but rather they emerge in solids as a result of these interactions. In this case, topological superconductors are believed to host quasiparticles named Majorana modes.
According to our best available descriptions of physics, every type of fundamental particle is accompanied by an antimatter counterpart, or antiparticle – which has the exact same mass, but exactly the opposite charge as its counterpart. If a particle ever crosses paths with its antiparticle in nature, the pair will immediately annihilate each other without any trace. However, as described by Italian physicist Ettore Majorana in the 1930s, some types of particles can theoretically behave like their own antiparticles.
Later on, physicists showed that this intriguing phenomenon wasn’t just a theoretical construct, and that Majorana quasiparticles can really emerge at topological superconductor interfaces. Since these quasiparticles arise as entangled pairs and are highly robust against the loss of quantum information, topological superconductors are now increasingly being explored for their potential use in quantum computing. However, one further challenge lies in the production of these materials, on scales large enough for them to host complex systems of qubits.
‘Robust quantum computers can enable exponentially faster calculations to solve very difficult and far-reaching problems, but their development rests on the design and discovery of new materials, such as topological superconductors.’
Molecular Beam Epitaxy
Although a variety of chemical compounds are predicted to behave as topological superconductors, their performance can vary widely depending on both their geometry and chemical composition. If the right approach isn’t taken when fabricating these materials, their physical properties could end up being detrimental to quantum computing applications. Through a careful approach to their fabrication, Dr Assaf, Dr Liu and their colleagues show how these useful properties can instead be enhanced.
Through their latest research, the team produced and measured the properties of a variety of candidate superconductor materials. As Dr Assaf explains, they fabricated these materials in the form of thin films. ‘This geometry is required for applications in quantum computing, but its impact on the fundamental properties of those superconductors may be detrimental or beneficial to their topological properties,’ he says. As a result, it is crucial to ensure that the film’s composition offers an optimal performance.
To fabricate the materials, the team used a technique named ‘molecular beam epitaxy’. This process takes place in an ultra-high vacuum, and begins by heating solid materials until they sublimate, forming a molecular gas. This gas is then fired in a molecular beam, and condenses onto a substrate – at a rate depending on the temperatures of the beam and the substrate. In time, this allows pure solid crystals to be built up atomic layer by atomic layer, until a solid thin film has formed. This process is illustrated in Figure 1 (right).
By carefully controlling the parameters of the technique, Dr Assaf, Dr Liu and their team developed superconducting films out of several different candidate materials, which they fabricated on top of a topological insulator. Afterwards, they searched for specific ingredients required to observe Majorana quasiparticles at the boundaries of the material.
As they discovered, the best performances arose in a superconducting compound containing a particular ratio of tin, indium, and tellurium – which could be easily scaled to the size of a large wafer. The crystal structure of this material is shown in Figure 2. This compound is especially desirable as it is non-toxic, non-radioactive, and compatible with several different types of semiconductor substrate – enabling more advanced device-based experiments to search for Majorana quasiparticles.
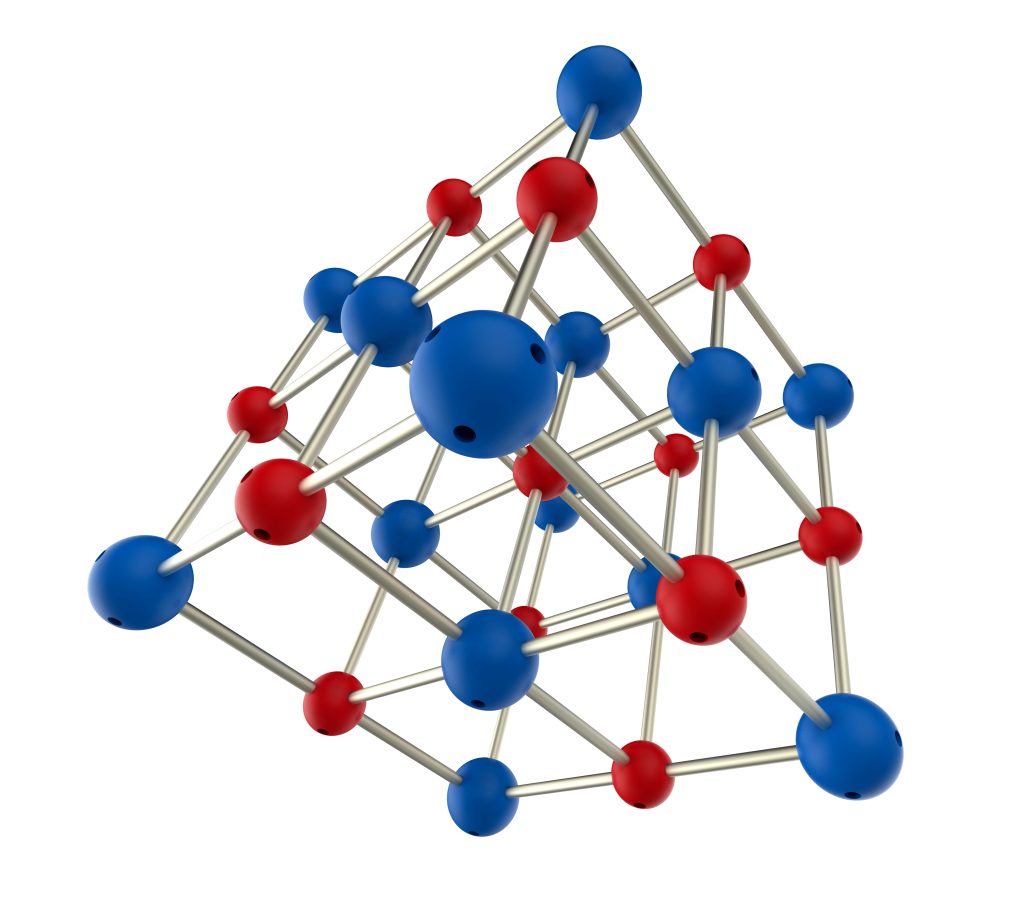
Figure 2: Cubic crystal structure of (Sn,In)Te.
Spin-orbit Coupling
As well as hosting superconductivity, Dr Assaf, Dr Liu and their colleagues also discovered intriguing patterns in the motions of the material’s electrons, which they hadn’t previously considered in their theoretical calculations. ‘Before it enters the superconducting state, the electrical conductivity of this material exhibits a strong coupling between the spin of the electron and its movement,’ Dr Assaf illustrates.
The spin Dr Assaf describes is not a physical rotation, but a key property of quantum particles, which relates to their magnetic moments. In this case, spin is intrinsically linked to the motions of atomic electrons, which follow circular orbits around positively-charged nuclei. Within an atom, electrons are strictly confined into discrete energy levels, and cannot exist in any state that lies between two energy levels. But when their spins and motions are coupled together in this way, it produces a ‘splitting’ in their energy levels. This splitting is an essential ingredient to achieve topological superconductivity.
The group is now collaborating with theoretical physicist Professor Yi-Ting Hsu from Notre Dame to further understand this material and identify new device-friendly topological superconductors. In future research, their results will lay the groundwork in the search for topological states that could be useful in quantum computing – where the quantum properties of Majorana quasiparticles could be manipulated for use as qubits.
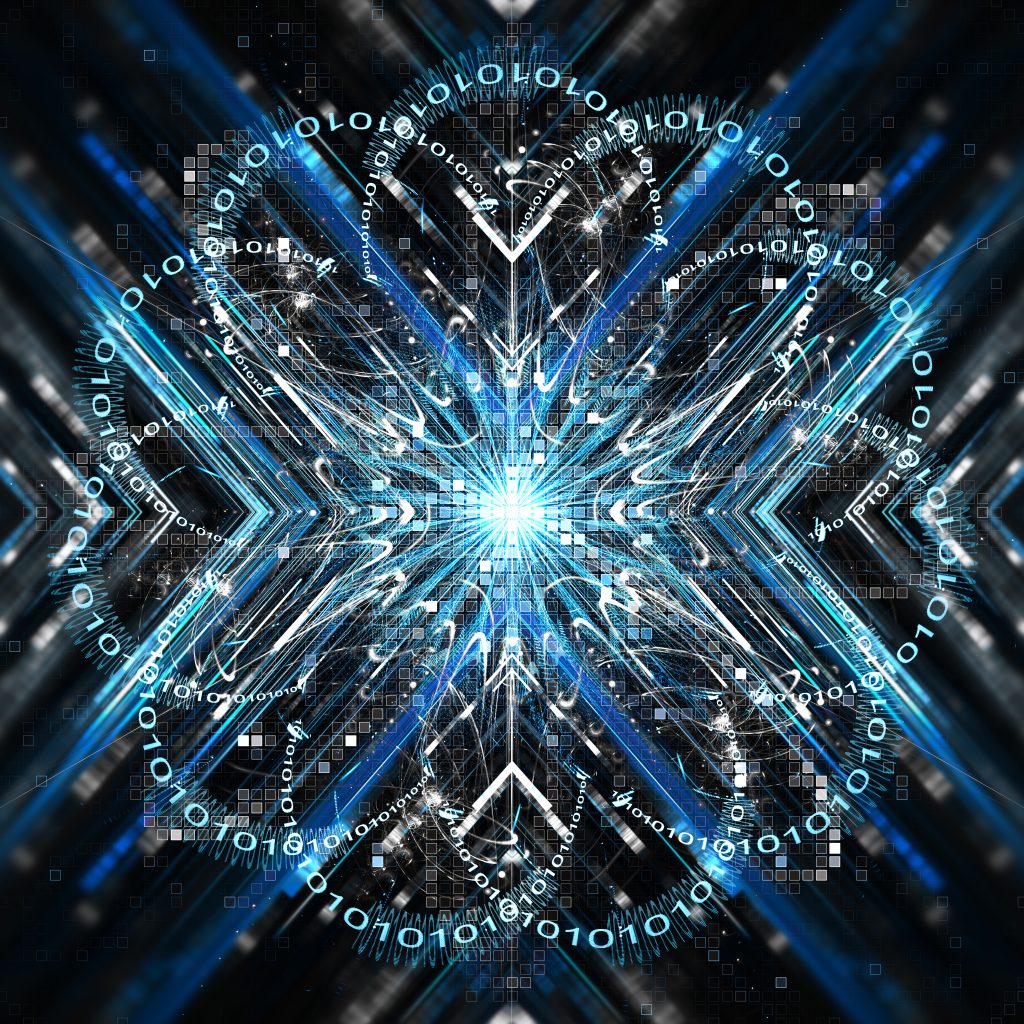
Artist’s representation of a qubit
Extending the Reach of Quantum Computing
If this could be achieved, it could one day lead to complex systems of qubits that are far more robust in the face of unexpected environmental changes than existing systems – allowing such systems to retain their quantum information far more reliably. This could lead to quantum computers that are far better suited to studying deeply complex natural systems – from the bonds and electrostatic forces found within large groups of atoms, to the deeply complex and interrelated process that play out within Earth’s atmosphere.
As Dr Assaf concludes, ‘Robust quantum computers can enable exponentially faster calculations to solve very difficult and far-reaching problems, but their development rests on the design and discovery of new materials, such as topological superconductors.’ In turn, the advantages of topological superconductors may one day be exploited for a diverse range of vitally important applications, which could have strong impacts on our everyday lives.
SHARE
DOWNLOAD E-BOOK
REFERENCE
https://doi.org/10.33548/SCIENTIA806
MEET THE RESEARCHERS
Dr Badih A. Assaf
Department of Physics
University of Notre Dame
Notre Dame, IN
USA
Dr Badih A. Assaf completed his PhD in Physics at Northeastern University, Boston, in 2014. Since 2018, he has worked as an Assistant Professor at the University of Notre Dame’s Department of Physics. Dr Assaf currently leads the department’s Topological Quantum Matter Group, which studies the properties of quantum material heterostructures and nanostructures, and their synthesis with molecular beam epitaxy. His research particularly focuses on the exotic ‘topological’ phases of these materials – which emerge at ultra-low temperatures.
CONTACT
E: bassaf@nd.edu
W: https://physics.nd.edu/people/badih-assaf/
Dr Xinyu Liu
Department of Physics
University of Notre Dame
Notre Dame, IN
USA
Dr Xinyu Liu completed his PhD in Condensed Matter Physics at the University of Notre Dame in 2003. In 2013, Dr Liu began his current position as a Research Associate Professor in the Department of Physics at this university. His main research interests include the structural, electrical, and optical properties of semiconductor alloys and their heterostructures, as well as the development and fabrication of semiconductor-based nanostructures.
CONTACT
E: xliu2@nd.edu
W: https://physics.nd.edu/people/xinyu-liu/
KEY COLLABORATORS
Dr Yi-Ting Hsu
Dr Michael Smith
Jiashu Wang
FUNDING
Division of Materials Research of the National Science Foundation – grant number: 1905277
FURTHER READING
J Wang, W Powers, Z Zhang, M Smith, BJ McIntosh, SK Bac, L Riney, M Zhukovskyi, T Orlova, LP Rokhinson, YT Hsu, X Liu, BA Assaf, Observation of Coexisting Weak Localization and Superconducting Fluctuations in Strained Sn1–xIn xTe Thin Films, Nano letters, 2022, 22, 2, 792–800.
L Riney, C Bunker, SK Bac, J Wang, D Battaglia, YC Park, M Dobrowolska, JK Furdyna, X Liu, BA Assaf, Introduction of Sr into Bi2Se3 thin films by molecular beam epitaxy, Journal of Applied Physics, 2021, 129, p.085107.
REPUBLISH OUR ARTICLES
We encourage all formats of sharing and republishing of our articles. Whether you want to host on your website, publication or blog, we welcome this. Find out more
Creative Commons Licence (CC BY 4.0)
This work is licensed under a Creative Commons Attribution 4.0 International License.
What does this mean?
Share: You can copy and redistribute the material in any medium or format
Adapt: You can change, and build upon the material for any purpose, even commercially.
Credit: You must give appropriate credit, provide a link to the license, and indicate if changes were made.
SUBSCRIBE NOW
Follow Us
MORE ARTICLES YOU MAY LIKE
Professor Aristides Marcano Olaizola | Innovation in the Production of Singlet Oxygen
A novel method for producing singlet oxygen via stimulated Raman scattering (SRS) offers an innovative alternative to traditional photosensitizer-based techniques, promising safer and more efficient applications in disinfection, cancer treatment, and beyond. Professor Aristides Marcano Olaizola at Delaware State University is driving innovation in this critical field.
Professor Darin Acosta | At the Frontier of High Energy Physics
Professor Darin Acosta’s research at the CMS experiment utilises advanced muon detection, sophisticated trigger systems, and machine learning to deepen our understanding of the Higgs boson and explore the potential existence of dark matter. Based at Rice University in the USA, Professor Acosta’s work has long-reaching implications that are fundamental to our understanding of the universe.
The Progressive Recovering of Einstein’s Determinism under Strong Interactions
Quantum mechanics relies on probabilities and uncertainties – for example, we cannot work out the outcome of a quantum system, but instead, we can suggest probabilities of certain outcomes. This has been troublesome for determinists, who instead believe that all outcomes are governed by a set of laws. Sir Professor Ruggero Maria Santilli from The Institute of Basic Research argues that if we extend our picture of quantum mechanics to his idea of hadronic mechanics, we can recover hidden variables and progressively recover determinism.
Professor Francisco Jurado | Taming the Dynamics of Nonlinear Partial Differential Equations
Controlling partial differential equations (PDEs) with parametric uncertainties is vital for system stability in science and engineering. Professor Francisco Jurado from Tecnológico Nacional de México (TecNM)/La Laguna addresses this challenging problem with a novel approach using adaptive backstepping boundary control for a one-dimensional modified Burgers’ equation. His innovative work tackles uncertainties in reactive and viscosity terms and considers Robin and Neumann boundary conditions, offering valuable insights into nonlinear PDE control.