Dr Bernhard Lüscher – Dr Patricia Korn | The Role of ADP-ribosylation in Innate Immunity
Innate immunity, our standing defence against viruses, bacteria, and other infections, is comprised of a vast network of biomolecules working in orchestrated balance to defend against pathogens. Researchers have theorised that ADP-ribosylation – where ADP-ribose groups are added to modify host and viral biomolecules – may constitute an active part of the innate immune system. Dr Bernhard Lüscher and Dr Patricia Korn from RWTH Aachen University lead a team that studies the role of ADP-ribosylation and viral function.
The Innate Immune Response
Immunity, how an organism resists infection, can be characterised as adaptive or innate. Innate immunity is the first line of defence, comprising a vigilant biological network which responds to threats without the need to learn from pathogens beforehand. This is because the innate immune system recognises biomolecules that are frequent in many different pathogens, so-called pathogen-associated molecular patterns – PAMPs (see Figure 1).
One group of biomolecules suggested to play an innate immune role are adenosine diphosphate (ADP)-ribosyltransferase proteins (ARTs). These are enzymes which transfer ADP-ribose groups to substrates, altering the way substrates interact with their surroundings. Many theorise that these enzymes are integral in innate immunity as key players in a billion-year arms race between viruses and their hosts.
If true, this would be instructive in the fight against viruses, offering an untapped reservoir of targets for therapies. Dr Bernhard Lüscher, Dr Patricia Korn, and their colleagues at RWTH Aachen University have reviewed the literature on ARTs to produce an assessment of their place in innate immunity.
The Viral Response Pathway
Our innate immune response begins with PAMPs, biomolecules particular to various pathogens, including viruses, impairing human health. Host cell receptors recognise PAMPs and promote the assembly of signalling complexes. These activate transcription factors that increase the expression of genes encoding different effector and signalling molecules, including interferons.
Interferons (IFNs) are proteins responsible for setting the innate immune system into motion. They engage cell surface receptors of the infected as well as neighbouring cells to kick off signalling events resulting in the activation of interferon-stimulated genes. This allows the spreading of information about contact with a pathogen, and subsequently, many IFN-stimulated genes are activated. Hundreds of these genes have been identified, many of which encode antiviral proteins (Figure 1).
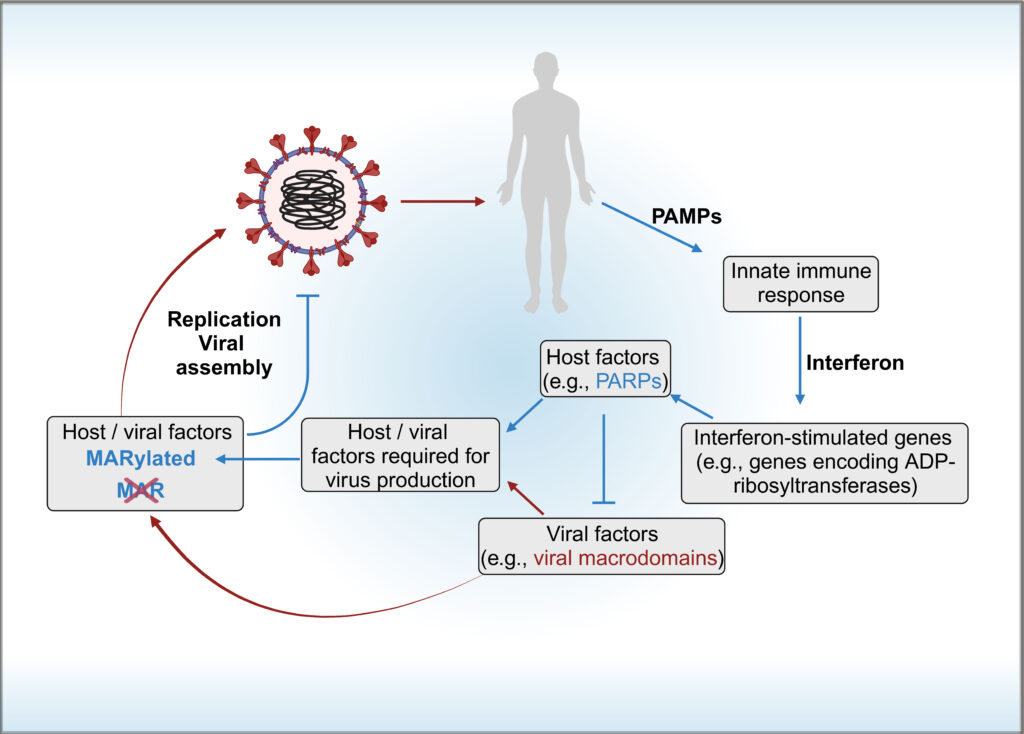
Figure 1
Adenosine Diphosphate Ribosyltransferases
Among the many genes regulated by interferons, some carry the information for ARTs. Their action, ADP-ribosylation, was uncovered in the 1960s and has since been found to influence DNA repair, stress response, and viral replication, as well as other cellular processes. ADP-ribosylation comes in two forms, mono- and poly-ADP-ribosylation (MARylation and PARylation, respectively). Some of the ARTs, including PARP 10, 12, 14, and 15, catalyse MARylation. Researchers have posited that this MARylation might be relevant to the innate immune system and Drs Lüscher and Korn and their team collated and summarised the evidence in support of this theory (see Figure 1).
Initial Observations
Early observations link individual PARP enzymes to interferon signalling. For example, it was observed that upon infection with Borrelia burgdorferi bacteria, the expression of PARPs 10, 12, and 14 was activated, with similar findings for coronavirus infections.
Another study found that among 10 animal species, 62 shared interferon-stimulated genes were upregulated by IFNs. These genes encode proteins thought to comprise the core innate immune response, several being PARPs encoding mono-ARTs. Also evaluated were ADP-ribosylhydrolases, enzymatic opposites to ARTs that cleave ADP-ribose groups and thus remove this modification from substrates. These were not upregulated by IFNs. This suggests that MARylation exerts an antiviral effect, upregulated during the immune response, while de-MARylation is not.
Understanding the dynamics of PARP expression as induced by IFNs and identifying PARP-substrate pairs is laborious, given their low expression and the difficulties in defining ADP-ribosylation sites in substrates. However, their evolutionary history points towards an antiviral role. PARP genes have undergone strong selection among primates, with rapid sequence adaptations thought to reflect an arms race against viral counter-adaptations. Sequence changes are particularly frequent in catalytic domains, the sites of substrate recognition and mono-ADP-ribosylation.
Role in Viral Propagation
Among the IFN-inducible ARTs, PARP13 is perhaps the best-studied antiviral ART protein. PARP13 has repressing activity against many viruses, but not all, suggesting specificity, yet does not contribute to ADP-ribosylation. PARP13 seems to recognise viral genetic materials, such as viral RNA, and bridge them with the exosome, a cellular structure which degrades RNA. Thus, PARP13 may directly eliminate some viral genomes, thereby inhibiting viral replication and propagation.
PARP13 also interacts with proteins necessary for translating the viral RNA into protein. This inhibits viral RNA from hijacking the cellular protein synthesis machinery for viral protein production. In addition to PARP13, PARPs 7, 10, and 12 also appear to repress translation, suggesting that this is an important mechanism of PARP proteins to interfere with viral production. PARP13 may additionally work in complex with PARPs 12 and 15 to MARylate the Dicer/RNA-induced silencing complex (RISC) to regulate RNA translation and, thus, protein biosynthesis. Moreover, PARPs 10, 12, 14, and 15 may MARylate components of stress granules, structures associated with immunity, protecting host RNAs from degradation. The conclusion from these and many other studies is that some PARP proteins have broad antiviral activities, both through their ability to MARylate substrates but also independent of their enzymatic activity, supporting the link between the IFN signalling system and the expression of PARP genes as part of innate immunity.
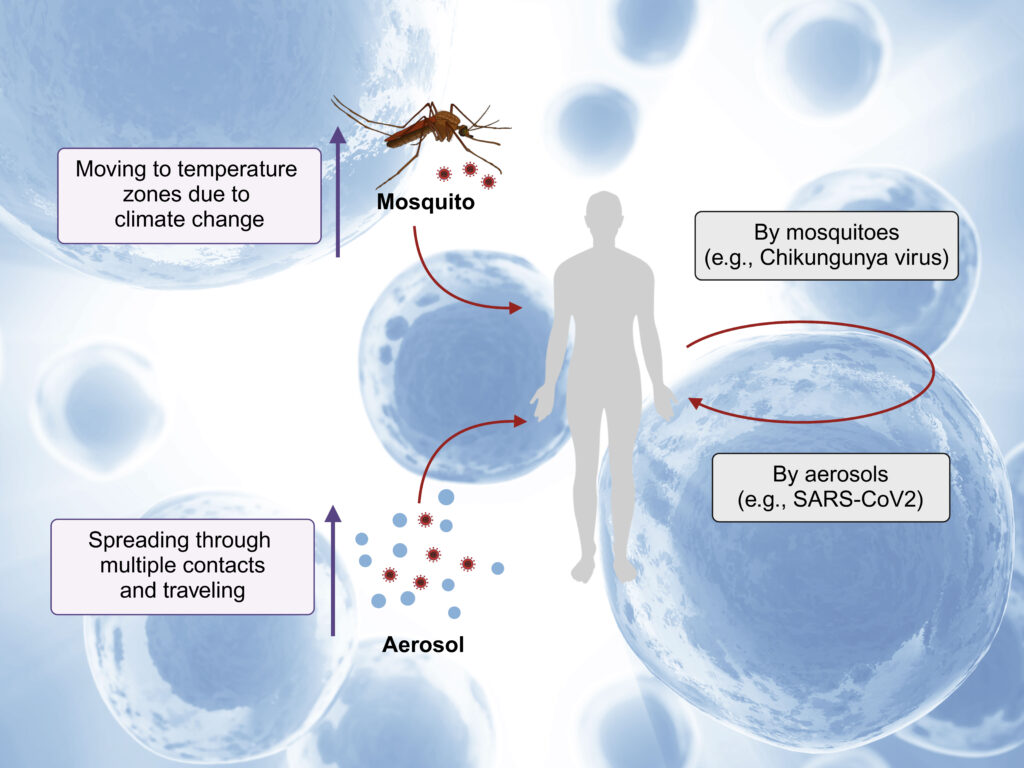
Figure 2
Viral Response
ARTs would be clearly evidenced to be involved in innate immunity if viruses were confirmed to develop defences against them. Dr Lüscher and Dr Korn point to viral macrodomains. These are structural elements of proteins which function as MAR-selective hydrolases (see Figure 1). There is evidence that viruses may also target PARPs directly, such as the avian influenza virus NS1 protein, which is suggested to induce PARP10 degradation. While there are only a few examples of the latter, i.e., direct targeting of PARPs, hydrolytically active macrodomains have been identified in different RNA viruses, which include SARS-CoV-2, Chikungunya virus, Venezuelan Equine Encephalitis virus, Hepatitis E virus, which are human pathogens. Their macrodomains’ selectivity for removing MARylation but not PARPylation further supports the notion that ARTs with MARylation activity possess antiviral functions.
Looking Forward
Dr Lüscher and Dr Korn draw several conclusions. Most critically, based on the findings that PARP genes are induced by interferons, but none of the genes that encode antagonising hydrolases, they suggest an antiviral role for MARylation. This appears consistent with the fact that many viruses possess macrodomains with de-MARylating activity, possibly to counter PARP functions that may affect both host and viral proteins.
But how would viruses which don’t encode active macrodomains deal with PARPs? They may develop other strategies, such as de-MARylating through so far unidentified domains or attacking PARPs directly. Some viruses control interferon signalling, potentially targeting PARPs at their source. As it stands, evidence strongly suggests that ADP-ribosylation is involved in innate immunity.
Confirmation would have widespread importance. Many relevant viruses are spread by mosquitos, for example, Chikungunya virus, or are transmitted by aerosols, such as SARS-CoV-2 (Figure 2). With international travel and global temperature rise, mosquitos and the pathogens they carry are becoming a concern for more and more people and virus spreading is accelerated.
As techniques develop, researchers expect a boost in MARylation substrates found and these findings to be put to therapeutic use. Already, computational screenings and homology analyses are finding molecules which potentially inhibit viral macrodomains, including suramin, dasatinib, and the remdesivir metabolite GS-441524. As such, this research opens a new front in the fight against viruses.
SHARE
DOWNLOAD E-BOOK
REFERENCE
https://doi.org/10.33548/SCIENTIA1139
MEET THE RESEARCHERS
Dr Bernhard Lüscher
RWTH Aachen University, North Rhine-Westphalia, Germany
Dr Bernhard Lüscher received his Diploma in Natural Sciences from ETH Zürich in 1981. He then studied at the University of Lausanne’s Institute of Biochemistry, where he earned his doctorate with the thesis ‘Structure, biosynthesis and maturation of Lyt-2/3 and Thy-1 antigens from mouse T lymphocytes’. He has held several academic posts in the USA and Germany, where he has spent his career researching molecular biology and biochemistry, teaching students, and publishing numerous papers. He has held positions on the review boards for Scientific Reports and the World Journal of Biological Chemistry, and is a member of the German Society for Biochemistry and Molecular Biology, the German Cancer Society, Life Sciences Switzerland, and the European Association for Cancer Research. Dr Lüscher was awarded the Sir Hans Krebs-Preis in 1996 for his medical research. He currently serves as Director of the Institute of Biochemistry and Molecular Biology at RWTH Aachen University.
CONTACT
E: luescher@rwth-aachen.de
W: https://www.ukaachen.de/kliniken-institute/institut-fuer-biochemie-und-molekularbiologie/institut/team/bernhard-luescher/
Dr Patricia Korn (maiden name Verheugd)
Aachen University, North Rhine-Westphalia, Germany
Dr Patricia Korn gained her Diploma in Biology at RWTH Aachen University in 2008. Her diploma thesis focused on the investigation of mouse herpesvirus 68 (MHV-68) and its modulation of specific antiviral immunity in mice. She then worked in microbiological diagnostics situated at the Institute of Microbiology at the University Hospital Aachen. In late 2009, she began work on her doctorate and defended her thesis titled ‘Mono-ADP-ribosylation by ARTD10 – a new modification in NF-κB signalling’. After this, she worked as a postdoctoral fellow at the Karolinska Institute in Sweden until returning to RWTH Aachen University in 2014. Here, she has published several papers on her research into the molecular biology of host-virus interactions while teaching students. She is a member of the German Society for Biochemistry and Molecular Biology, the German Society of Virology and InfectNet. Dr Korn’s work uncovers the emerging mechanism of mono-ADP-ribosylation as a posttranslational modification and its implications for host-pathogen conflict. She now serves as Group Leader at the RWTH Aachen University Institute of Biochemistry and Molecular Biology.
CONTACT
E: pkorn@ukaachen.de
W: https://www.ukaachen.de/kliniken-institute/institut-fuer-biochemie-und-molekularbiologie/institut/team/patricia-korn-geb-verheugd/
KEY COLLABORATORS
Dr Carsten Bolm, RWTH Aachen University
Dr Christine Goffinet, University of Liverpool
Dr Michael Hottiger, University of Zurich
Dr Stefan Knapp, Goethe University of Frankfurt
Dr Andreas Ladurner, Ludwig Maximilians University of Munich
Dr Lari Lehtiö, University of Oulu
Dr Giulia Rossetti, Forschungszentrum Jülich
FUNDING
Open access funding by Projekt DEAL
Deutsche Forschungsgemeinschaft DFG
The Wilhelm-Sander Foundation
The Volkswagen (VW) Foundation
The START program of the Medical School of the RWTH Aachen University
FURTHER READING
K Biaesch, S Knapp, P Korn, IFN-Induced PARPs—Sensors of Foreign Nucleic Acids?, Pathogens, 2023, 12(3), 457. DOI: https://doi.org/10.3390/pathogens12030457
S Krieg, F Pott, L Potthoff, et al., Mono‐ADP‐ribosylation by PARP10 inhibits Chikungunya virus nsP2 proteolytic activity and viral replication, Cellular and Molecular Life Sciences, 2023, 80, 72. DOI: https://doi.org/10.1007/s00018-023-04717-8
B Lüscher, I Ahel, M Altmeyer, et al., ADP-ribosyltransferases, an update on function and nomenclature, The FEBS Journal, 2021, 289(23), 7399–7410. DOI: https://doi.org/10.1111/febs.16142
B Lüscher, M Verheirstraeten, S Krieg, P Korn, Intracellular mono‐ADP‐ribosyltransferases at the host–virus interphase, Cellular and Molecular Life Sciences, 2022, 79, 288. DOI: https://doi.org/10.1007/s00018-022-04290-6
M Naddaf, Mosquito-borne diseases are surging in Europe, Nature, 2024, 633, 749. DOI: https://doi.org/10.1038/d41586-024-03031-y
JGM Rack, L Palazzo, I Ahel, (ADP-ribosyl)hydrolases: structure, function, and biology, Genes & Development, 2020, 34, 263–284. DOI: https://doi.org/10.1101/gad.334631.119
P Verheugd, AH Forst, L Milke, et al., Regulation of NF-kB signaling by the mono-ADP-ribosyltransferase ARTD10, Nature Communications, 2013, 4, 1683. DOI: https://doi.org/10.1038/ncomms2672
REPUBLISH OUR ARTICLES
We encourage all formats of sharing and republishing of our articles. Whether you want to host on your website, publication or blog, we welcome this. Find out more
Creative Commons Licence (CC BY 4.0)
This work is licensed under a Creative Commons Attribution 4.0 International License.
What does this mean?
Share: You can copy and redistribute the material in any medium or format
Adapt: You can change, and build upon the material for any purpose, even commercially.
Credit: You must give appropriate credit, provide a link to the license, and indicate if changes were made.
SUBSCRIBE NOW
Follow Us
MORE ARTICLES YOU MAY LIKE
Dr Maka Tsulukidze – Dr David Reardon | After the Loss: Exploring Cardiovascular Risks Linked to Pregnancy Loss
Carrying a pregnancy is associated with numerous health problems, but little is known about the impact of pregnancy loss on heart health. Dr Maka Tsulukidze from the Florida Gulf Coast University, Dr David Reardon, based at the Elliot Institute, and Dr Christopher Craver at the Charlotte Lozier Institute in Arlington, conducted research into the adverse effects of pregnancy loss on heart function and health. In particular, their research focused on the impact of pregnancy loss on the risk of cardiovascular diseases.
Dr Zhi-yong Huang | The Scientific Hepatectomy: Pioneering Approaches for Tackling Liver Cancer
Cancer research is an ever-evolving field of science fuelled by the pressure of the ongoing need to find new approaches to tackle this killer. Dr Zhi-yong Huang is based at the Tongji Medical College at Huazhong University of Science and Technology in China. He works tirelessly to develop novel treatments for diseases of the liver and biliary system, with a particular focus on liver cancer surgery. He has pioneered methods to optimise the treatment of this type of cancer.
Dr Denise Faustman | An Unexpected Ally in the Fight Against Diabetes: The BCG Vaccine
Diabetes is a widespread health concern affecting millions worldwide. Recent research suggests an unexpected ally in the fight against this disorder: the Bacille Calmette-Guérin (BCG) vaccine. Originally developed to prevent tuberculosis, BCG is now showing promise in managing and potentially preventing type 1 diabetes. Dr Denise Faustman from Massachusetts General Hospital (MGH) and Harvard Medical School is leading groundbreaking studies that are reshaping our understanding of diabetes treatment and prevention.
Dr Michael Hicks | Creating Skeletal Muscle from Stem Cells
Recognising the precise steps involved in the differentiation process of stem cells into various cell types is crucial to regenerative medicine. Researchers face the specific challenge of how to fine-tune and optimise protocols for directing stem cells to differentiate into specific cell lineages. As such, the directed differentiation to skeletal muscles has not yet advanced to the clinical trials stage. Dr. Michael Hicks from the School of Medicine at the University of California, Irvine, is working to change this with his extensive studies into the transition of stem cells into skeletal muscles.