Dr Sam Poppe | New Approaches to Understanding Volcanic Activity on Planetary Bodies
Understanding how subsurface magmatic activity affects the Earth’s crust is crucial to accurately forecasting volcano eruptions. Dr Sam Poppe and his team at the Space Research Centre of the Polish Academy of Sciences have developed a multimethod approach combining scaled laboratory models, fieldwork, observations of other planetary bodies, and numerical models to determine the effect of magma emplacement dynamics on the crusts of planetary bodies like the Earth, Moon, and Mars. By progressing the understanding of the mechanics of volcanoes, their work can improve the prediction of eruptions on Earth and understanding of past volcanic activity on our surrounding moons and planets.
Understanding Volcano Hazards
Volcanoes are openings on a planet’s surface where lava, volcanic particles like ash, and gases escape from underneath. The Earth is composed of a solid iron inner core, liquid iron outer core, mostly solid rock mantle layer, and solid crust. Where conditions in the mantle or crust favour the presence of molten rock, patches of extremely hot liquid rock (magma) form there. That magma can push to the crust and later solidify at shallower levels or ascend to the surface and cause a volcanic eruption.
Understanding magma reservoir dynamics and subsurface movements under the crust is essential to building an accurate picture of the timing and severity of unrest episodes and eruptions, enabling us to forecast and critically, mitigate the hazards that volcanic activity presents to society.
Magma Dynamics Under a Planet’s Surface
The movement of magma can affect a planet’s surface in many ways. When magma pushes upwards through the crust of a rocky planetary body, it often cools and solidifies before it reaches the surface.
In this case, the horizontally spread magma pries rock layers apart and may become a thicker batch of magma with a dome-shaped top and a flat base, known as a laccolith. Scientists mostly study laccoliths on Earth through field observations at inactive and exposed volcanoes.
Fractured Craters on the Moon
Investigating laccoliths on the well-preserved and uneroded surfaces of other planetary bodies allows scientists to better understand active volcanoes on Earth. Fracturing and bulges have been observed on the floors of impact craters on rocky bodies like the Moon or Mars, which have been attributed to shallow laccoliths under the surface. However, a lack of subsurface data means that their origins are still poorly understood. As floor-fractured craters on the Moon’s surface are only observable from space, researchers must rely on models to quantitatively study crust deformation.
Scientists have developed various models to understand the processes that occur under the surfaces of planetary bodies. However, these models assume that the rock layers there are elastic (materials that do not permanently deform) and exhibit a linear relationship between stress (the force applied to a material over a particular area) and strain (the material deformation caused by the applied force).
As magma-induced deformation observed on Earth is often non-elastic as permanent rock layer deformation occurs, and the architecture of magma intrusions can be varied and complex, this leads to a mismatch between the available models and real effects, and makes it difficult to form an accurate picture of magma intrusion. Few researchers have developed numerical models to determine how non-elastic magma-induced changes affect surface deformation patterns, and so far, none of these have been applied to floor-fractured Lunar and Martian craters.
Small Volcanoes in the Laboratory
Dr Sam Poppe and his team at the Space Research Centre of the Polish Academy of Sciences seek to understand surface displacement and dynamic fracturing during magma intrusion in a quantitative manner.
A particular difficulty is that current models of volcano deformation fail to take into account the sheer complexity of the rocks that comprise the planet´s crust and, indeed, volcanoes. Dr Poppe notes that this can lead to considerable underestimates of the volume and position of magma below volcanoes that may potentially erupt, as well as an inadequate understanding of the fracture networks created by magma below volcanoes.
During his PhD in Brussels, Dr Poppe initially described magma behaviour and rock displacement in controlled laboratory environments using a novel quantitative approach that combined scaled physical models with medical-quality X-ray imaging over time. Central to their method was the idea of moving from qualitative to quantitative experimental simulations of magmatic processes.
These fascinating sandbox experiments – using sand-and-plaster and golden syrup as analogues for host rocks and magma – showed that different properties of ‘host’ rocks lead to different geometries of magma intrusions. The team pioneered a method of imaging the experimental ‘unusual patients’ in 3-D over time (i.e., 4-D) to quantify their observations.
Mapping Magma Dynamics Over Time
Dr Poppe’s laboratory-based approach provided an inside-view of magma intrusion in a granular crust rock material, allowing the team to quantify intrusion-induced 3-D changes and strain over time. They observed the formation of thick hidden domes and thin dikes in their scaled models, and quantified host rock deformation and intrusion shape for various magma intrusion speeds and crust heights.
These experiments led to clear insights into shallow crust deformation in a laboratory setting. The next step was to use a combination of numerical simulations and field observations.
The DeMo-Planet Modelling Project
Dr Poppe and his team at the Space Research Centre – Polish Academy of Sciences needed to model magma-induced fracture dynamics accurately. A particle-based numerical model allowed the researchers to simulate how rocks fracture and displace around a growing magma body in the upper crust of a rocky planetary body. Fracture dynamics were compared for rocks with a range of mechanical properties and from different gravitational conditions to account for variations in rocks on the Moon, Mars and Earth.
Dr Poppe explains that rocks on the Moon and Mars have already been heavily fractured by meteorite impacts. To investigate the crustal deformation caused by magma intrusion on terrestrial planetary bodies, the team used a combination of laboratory tests, fieldwork and numerical simulations.
The main aim of the DeMo-Planet project was to determine how deformation induced by shallow magma intrusions on terrestrial bodies like the Moon are affected by the properties of the crust rock, fracture networks and chemical reactions that involve heat. These findings could then be used to provide a more realistic modelling approach to understanding magma dynamics in Earth’s active volcanoes.
The team chose parameters for their model using geological observations. ‘We use field observations and rock samples from quarries in Permian-age intrusions in the Sudetes mountains of Southwest Poland to calibrate our numerical models and validate them, so we can then better apply them to studying magma intrusions on the Moon and Mars, where we cannot obtain close-range field observations,’ explains Dr Poppe.
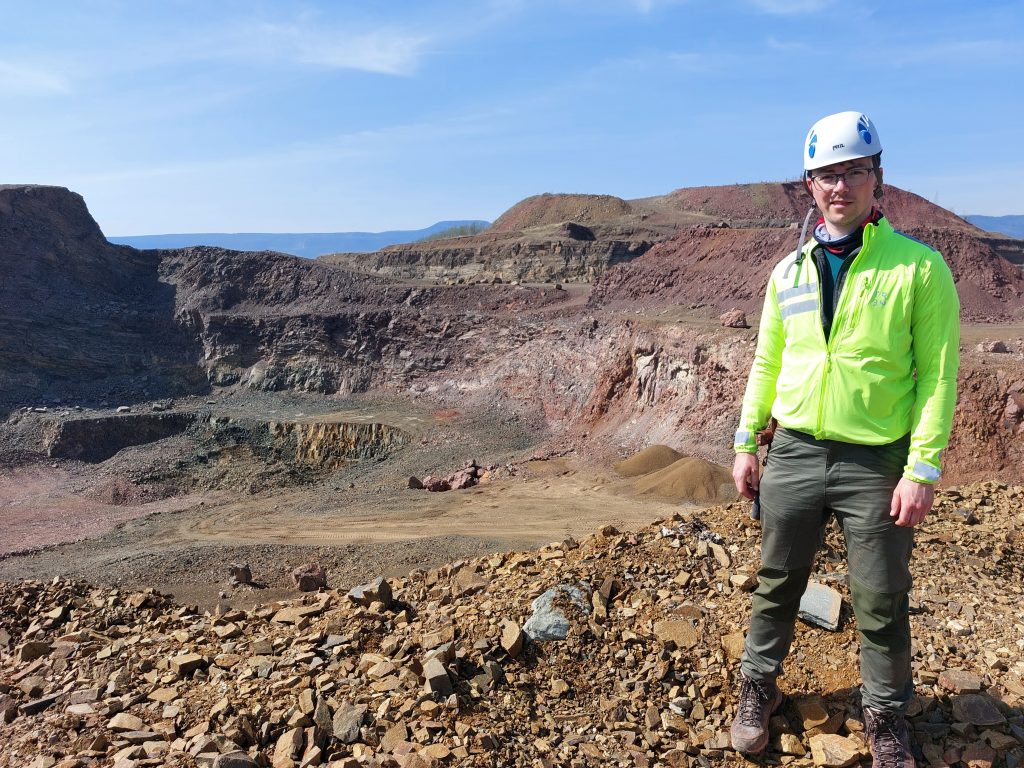
Credits: Sam Popp
Mapping Minerals and Mechanical Properties
The DeMo-Planet project relied on the collaborative efforts of many researchers working in both computational and laboratory settings, as well as fieldwork. The researchers calibrate rock strengths for numerical laboratory experiments using experimentally obtained values for natural rocks. Project scientists characterise fracture networks in the crust using a digital environment, and also map surface deformation on the Moon and Mars.
Other researchers within the project collected volcanic and sedimentary rock samples to compile a database of their mechanical properties. Rock samples were analysed in university laboratories in Brussels and Strasbourg to discover their exact composition and structural integrity.
Realistic Non-Elastic Numerical Models
The experimental aspects of the project are complemented by numerical simulations to gain a more complete idea of crust rock dynamics. Dr Poppe and postdoctoral researcher Dr Alexandra Morand used the Discrete Element Method (DEM; a numerical approach to computing the motion and effects of a large number of small particles) to study deformation and dynamic fracturing while also visualising stress and strain below the surface of a planetary body’s crust.
The team’s DEM model simulates magma emplacement in a homogeneous crust rock, allowing for magma intrusion. Key experimentally obtained information on the rock properties and fracture networks was used to fine-tune the model, and the researchers also studied the effect of using a more realistic heterogeneous crust medium. In this way, the team could model dynamic magma intrusion in weakened heterogeneous planetary crusts.
Deformation and Dynamic Fracturing Above Laccoliths
The team used their multimethod approach to model dynamic magma intrusion in a particle-based simulated crust. After calibrating the rock strength parameters needed for their simulations through laboratory experiments, they systematically investigated the effects of intrusion depth and various types of rock strength parameters on strain, stress and fracturing of the crust.
High crust stiffness (resistance to bending) led to widely distributed fracturing, while low stiffness resulted in a concentrated and more central fracturing pattern. These findings helped the team to understand fracture distribution patterns above laccolith intrusions.
Modelling Craters on the Moon and Mars
Turning their focus to craters on the surfaces of planetary bodies, the team modelled dynamic fracturing and displacement in a crust during the simulated inflation of a laccolith intrusion. The researchers also studied the effect of gravity on strain, stress, and fracturing above inflating laccoliths on the Moon, Mars, and Earth.
Dr Poppe and his team used their multimethod approach to better understand how the mechanical properties of planetary crusts control magma intrusion. This helped them to understand the fracture distribution patterns above laccolith intrusions in the shallow crusts of these rocky planetary bodies.
The method also provides other scientists with a quantitative approach to investigating the dynamic fracturing, magma intrusion and crust deformation dynamics that are essential to understanding the mechanisms underpinning fracture and deformation of crater floors and the development of hydrothermal systems on bodies such as the Moon or Mars.
Future of Volcano Modelling
Future research in volcano dynamics could include broadening and diversifying experimental methods and incorporating multidisciplinary analysis approaches to simultaneously model volcanic dynamics in laboratory settings. Another way to improve modelling accuracy would be to increase the sizes of experiments in dedicated warehouses or outdoor laboratories to approach the real scales of volcanic processes. The team could then use the information obtained from these experiments to guide, calibrate, and validate advanced numerical models.
Dr Poppe hopes that experiments and numerical models can be combined to bridge gaps in our knowledge of volcanic activity, guiding future studies into the Earth’s crust and the surfaces of other terrestrial bodies. By advancing insights into magma unrest in active volcanoes on Earth and passive ones beyond, this fascinating work can inform volcano monitoring efforts, eruption prediction, and future planetary exploration missions.
SHARE
DOWNLOAD E-BOOK
TRANSLATION
REFERENCE
https://doi.org/10.33548/SCIENTIA999
MEET THE RESEARCHER
Dr Sam Poppe,
Space Research Centre,
Polish Academy of Sciences,
Warsaw,
Poland
Dr Sam Poppe gained his bachelor’s and master’s degrees in geology from Ghent University. He then moved to the Vrije Universiteit Brussel as a staff scientist focusing on geo-risk in Central Africa. Dr Poppe obtained his PhD at the same institution, and moved to Pennsylvania State University as a Fulbright-BAEF postdoctoral fellow to investigate laboratory volcano geodesy. Dr Poppe also worked on the MagmaTect-4D project at the Université libre de Bruxelles before moving to the Space Research Centre of the Polish Academy of Sciences in 2021 as principal investigator on the DeMo-Planet magma intrusion modelling project. Dr Poppe focuses on the structural deformation of volcanic edifices on terrestrial planetary bodies to better inform volcanic eruption forecasting on Earth and help reconstruct the volcanic histories of other terrestrial planetary bodies. He is also a member of the Lunar Geology Orbiter science team funded by the European Space Agency in 2023. In the DeMo-Planet project, Dr Poppe combines various methods to model magma-induced dynamic surface fracturing on the Moon and Mars.
CONTACT
Centrum Badań Kosmicznych PAN, Bartycka 18A, 00-716 Warszawa, Poland
sam35poppe@gmail.com / sampoppe@cbk.waw.pl
sampoppevolcano.wixsite.com/sampoppe
FURTHER READING
A Morand, S Poppe, C Harnett, et al., Fracturing and dome-shaped surface displacements above laccolith intrusions: Insights from Discrete Element Method modelling, Journal of Geophysical Research – Solid Earth, 2024, JGRB_56627. DOI: https://doi.org/10.1029/2023JB027423
S Poppe, A Morand, CE Harnett, et al., A new model of deformation and dynamic fracturing above laccolith intrusions, EGU General Assembly 2023, Vienna, EGU23–1337. DOI: https://doi.org/10.5194/egusphere-egu23-1337
S Poppe, A Morand, A Cornillon, C Harnett, Modeling of surface displacement and dynamic fracturing during magma emplacement at floor-fractured craters on the Moon and Mars, EGU General Assembly 2023, Vienna, EGU23–16365. DOI: https://doi.org/10.5194/egusphere-egu23-16365
S Poppe, JT Gilchrist, ECP Breard, et al., Analog experiments in volcanology: towards multimethod, upscaled, and integrated models, Bulletin of Volcanology, 2022, 84, 52. DOI: https://doi.org/10.1007/s00445-022-01543-x
S Poppe, EP Holohan, O Galland, et al., An Inside Perspective on Magma Intrusion: Quantifying 3D Displacement and Strain in Laboratory Experiments by Dynamic X-Ray Computed Tomography, Frontiers in Earth Science, 2019, 7, 62. DOI: https://doi.org/10.3389/feart.2019.00062
key collaborators
Dr Alexandra Morand, Space Research Centre, Polish Academy of Sciences (now at Bristol University, UK)
Professor Dr Hab Daniel Mège, Space Research Centre, Polish Academy of Sciences
Professor Dr Hab Marek Awdankiewicz, University of Wroclaw
Dr Claire Harnett, University College Dublin
Professor Dr Michael Heap, University of Strasbourg
Professor Dr Karen Fontijn, Université libre de Bruxelles
Professor Dr Matthieu Kervyn, Vrije Universiteit Brussel
Dr Björn Nyberg, University of Bergen
Dr Petr Brož, Institute of Geophysics, Czech Academy
of Sciences
Prof Dr Michael Petronis, New Mexico Highlands University
Professor Dr Christelle Wauthier, Pennsylvania State University
FUNDING
Norwegian Financial Mechanism
Polish National Centre for Science
Polish National Agency for Academic Exchange
Vocatio Foundation
REPUBLISH OUR ARTICLES
We encourage all formats of sharing and republishing of our articles. Whether you want to host on your website, publication or blog, we welcome this. Find out more
Creative Commons Licence (CC BY 4.0)
This work is licensed under a Creative Commons Attribution 4.0 International License.
What does this mean?
Share: You can copy and redistribute the material in any medium or format
Adapt: You can change, and build upon the material for any purpose, even commercially.
Credit: You must give appropriate credit, provide a link to the license, and indicate if changes were made.
SUBSCRIBE NOW
Follow Us
MORE ARTICLES YOU MAY LIKE
RK Pete Wade | Seeing the Wood for the Trees – A Better Way to Measure Forest Density
In the world of forestry, understanding how densely packed trees are in a given area is crucial for effective forest management. However, traditional methods for measuring this ‘stand density’ have limitations. Now, a new approach developed by an independent researcher offers a more nuanced and accurate way to assess forest density across different species and environments.
Dr Peter Gent | Climate ‘Normals’ Are Anything But Normal In Weather Forecasting
Weather forecasters and meteorologists have long used the term ‘climate normal’ to describe average temperatures, but this seemingly innocuous phrase might be causing widespread public misunderstanding. New research suggests that describing temperature data as ‘normal’ leads many people to incorrectly assume these values represent the most common or expected temperatures when, in reality, actual temperatures regularly deviate significantly from these averages.
Dr Yassine Ait Brahim | When the Sahara Grew Green: Constraining the ‘Green Sahara’ Across Time and Space
Understanding the dynamics of Earth’s ancient climate is an important avenue of research. By examining past climate variability, we can improve our understanding of natural climate cycles and factors influencing current climate change. Dr Yassine Ait Brahim of the University Mohammed VI Polytechnic (UM6P) in Morocco worked with colleagues to analyse isotopic data recovered from cave mineral deposits in Northwest Africa. This exciting research is focused on the precipitation history of the Sahara Desert, which, perhaps astonishingly, had periods of vegetation cover and mega-lakes.
Dr Festus O Amadu | The Forests of Liberia: A Pathway to Poverty Alleviation and Food Security
In Liberia, half of the population lives within 2.5 kms of a forest. Many households rely on these delicate ecosystems to support themselves. A recent study by Dr Festus O Amadu at Florida Gulf Coast University and Dr Daniel Miller at the University of Notre Dame sees sustainable forest management as a positive force in improving livelihoods and environmental outcomes in Liberia. The authors have produced a landmark national analysis of how participation in the ‘forest sector’ impacts Liberian households – providing policymakers with the evidence they need to prioritise development programmes.