Professor Dominique Durand – Plugging into the Nervous System
New advances in neural engineering have led to devices that can be operated using the nerves of the user, but the effectiveness and safety of these devices over long periods of use is a key concern. Professor Dominique Durand, Director of the Neural Engineering Center at Case Western Reserve University, leads a team of scientists looking to improve neuroprosthetics through developing new methods of interfacing with the nervous system.
New Advances for Amputees
Until recently, the concept of prosthetic limbs that can be controlled by the human nervous system was confined to the world of science fiction. Recent advances have given us prostheses that can interact directly with the nervous system, giving the user back the use of an arm or a leg, or a fully articulated hand with each finger individually controllable.
With more than 1 million limb amputations taking place globally each year, the need for safe and effective prosthetics is critical. The greatest challenges facing fully functioning, user-controlled prosthetics are reliability and safety, and ensuring that the prosthesis responds to real, measurable nerve signals and can be attached over a long period of time without causing damage to the nerves.
One of the researchers addressing these challenges in a novel way is Professor Dominique Durand, E L Lindseth Professor of Biomedical Engineering and Neurosciences and also Director of the Neural Engineering Center at Case Western Reserve University, USA.
Professor Durand and his team work in the emerging field of neural engineering, a new discipline at the intersection of neuroscience, neurology, and engineering. Neural engineering is an interdisciplinary research area that involves applying engineering techniques to the study and manipulation of the nervous system, with the aim to better understand how the nervous system works and provide new treatments for neurological dysfunction. Fields within neural engineering include, but are not limited to, the development and use of neural interfaces for neuroprosthetics, such as neural controlled artificial limbs for amputees or altering neural activity by direct stimulation of the central or autonomic nervous system (neuromodulation).
Professor Durand’s work aims to address two distinct neurological problems; the first is how to control disorders of the central nervous system such as epilepsy through electrical stimulation of the brain. The second is how to interface with the peripheral nervous system in order to restore function in patients with autonomic imbalance and give voluntary control of artificial limbs to amputees using neural prosthetics.
The Problems with Prosthesis
The majority of user-controlled prosthetics have utilised electromyography (EMG) pads that attach to the skin and detect the electric activity of existing muscles, generating signals that can then be sent to a prosthetic limb and direct it to move. However, patients with muscle damage to the upper-arm or with upper-arm amputations may not have muscles available to control the prostheses.
More recent prosthetic devices can chronically attach to the user by interacting directly with the nervous system. Instead of EMG electrodes, these devices use probes that are implanted into the body and wrap around nerves, called cuff or wire hooks electrodes. These are limited by their ability to differentiate real nerve impulses against background noise which can be caused by static as the cuffs move against the nerves.
Cutting edge research in prosthetics has led to probes that can enter inside the nerve itself and detect nerve impulses directly. These are much better at determining which nerve impulses are ‘real’, but their safety is as-yet untested when used long-term in implants.
A breakthrough from Professor Durand and his group in 2017 led to the development of a novel method of allowing technology to interface directly with the nervous system. They hypothesised that probes have had limited previous success use due to their stiffness. Nerve fibres are very flexible, and the team identified the need for a flexible probe that would match the mechanical properties of the nerve to prevent the probe from moving and causing irritation over the lifetime of the implant.
The Carbon Connection
To make a much more flexible probe, Professor Durand’s team formulated a probe composed of strings made of nanometre-wide strands called carbon nanotubes (CNTs). The original idea was to design an axon-like probe with dimensions and flexibility similar to other axons near the electrode. CNTs are a form of carbon, like graphite and diamond, where molecules of carbon are arranged into long tubular structures. The team spun the CNTs into an extremely thin multi-stranded yarn much thinner than the diameter of a nerve. The thinness of the probe meant that it could be implanted into the nerve with the aid of a microscopic metal needle called a microneedle.
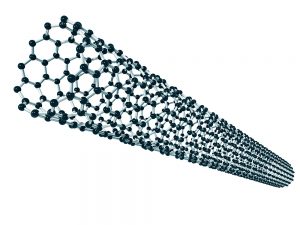
Illustration of a carbon nanotube
Professor Durand’s team tested the flexibility of the new yarn constructed from CNTs using a technique called atomic force microscopy and found that it was much more conductive and flexible than the platinum-iridium wire usually used for implants, with flexibility closely matching that of a nerve fibre. The team concluded this material would be perfect for the basis of a neural interface.
To test the probe, Professor Durand’s team first implanted the probe into the vagus nerve of a rat. The vagus nerve is situated in the neck and is a major conduit for nervous communication between the brain and the body. It receives sensory information from the organs of the body, including the heart, lungs, and the gut. The group measured the signals generated from the vagus nerve and a large range of signals coming the various organs.
Professor Durand’s team then implanted the probe in another major nerve of the neck called the glossopharyngeal nerve. This nerve transmits impulses from the carotid sinus which senses oxygen and pressure regulation. The group induced a hypoxic event in the rat, and then measured the nerve activity using the probe. They found they could detect the two types of signals produced by the carotid sinus that give information to the brain about the blood oxygen level and blood pressure.
Precision and Safety
Once the team knew the probe was effective in detecting nerve impulses, they went on to test whether the probe could detect impulses from specific parts of the nerve. Nerves are made up of bundles of tiny nerve fibres called axons that carry various signals. The carbon nanotube probe is about the size of one of these axons, which allows several probes to be implanted within very small nerves.
This approach allowed the team to measure the activity of individual bundles of axons within the nerve itself. They found when the rats were exposed to hypoxia, two different probes in the same nerve gave very different responses, indicating that each probe could selectively record different neural signals, such as baroreceptors and chemoreceptors. This shows that a greater and more accurate level of selectivity in detecting nerve impulses can be obtained using these tiny probes.
Finally, Professor Durand’s group tested their hypothesis that the flexible fibres would cause less irritation after being implanted in the body over a long period of time. In other systems, long-term insertion of a probe into a nerve can cause a problem where the body’s immune system responds to irritation and inflammation at the site of implantation. The foreign body response can cause a thickening of the connective tissue and scarring, which can come between the probe and the nerve leading to a significant decrease in recorded signal amplitudes. This effect can interfere with the normal function of a user-controlled prosthesis, and may even cause damage to the surrounding tissue.
The team found that chronic implantation of CNTs generated only minimal amount of inflammation around the site of implantation. The group looked at the area where the probe has been inserted and found there were few immune cells around the site of implantation, indicating a limited inflammatory reaction in response to the Carbon Nanotube probe.
New Frontiers in Neural Interfaces
Professor Durand’s team’s findings confirmed their hypothesis that probes made from CNTs would be more suitable for chronic implants, causing less damage, and maintaining signal for a longer period. The group hopes that these findings will pave the way for more effective carbon nanotube-based controllable prosthetics. Future work by Professor Durand and his group will also focus on the development of a new neural control system for a prosthetic arm using their novel technologies.
Critically, not only was the CNT yarn system capable of detecting nerve impulses, it was also able to stimulate nerves. Whereas detecting nerve impulses is key in designing artificial prostheses, the ability of the probe to both detect and stimulate nerves could mean it could be used to even repair nerves damaged by neurological injuries, such as strokes and spinal injury. Each year, over 100,000 people in the UK suffer strokes. It is the leading cause of disability in the UK, with two-thirds of stroke survivors suffering some sort of prolonged disability caused by damage to the nervous system.
Professor Durand’s new technique opens up a host of new opportunities to combat some of the most complex and impactful problems in neurology today. Science fiction no longer, new findings from Professor Durand’s lab could lead the way to new, more effective and stable prosthetics and give us new ways of treating disorders of the nervous system.
Reference
https://doi.org/10.33548/SCIENTIA407
Meet the researcher
Professor Dominique Durand
Departments of Biomedical Engineering, Neurosciences, Physiology and Biophysics, Electrical Engineering
Neural Engineering Center
Case Western Reserve University
Cleveland, OH
USA
Professor Dominique Durand received his PhD in Electrical Engineering from the University of Toronto. On completion of his doctorate in 1982, Professor Durand moved to Cleveland, USA to take up an Assistant Professor position at Case Western Reserve University, Ohio where he is currently the E L Lindseth Professor of Biomedical Engineering. Professor Durand’s research is focused on solving problems in the central and peripheral nervous systems through the disciplines of neural engineering, computational neuroscience, and neurophysiology. In particular, he is working on how to understand and restore neural function in both the central and peripheral nervous systems, investigating how applied currents could be used to control seizures in patients with epilepsy, and improving user-controlled prosthetics. In 2000, he was appointed Director of the Neural Engineering Center, a multidisciplinary institute dedicated to research at the interface between neuroscience and engineering. He is the founder and editor-in-chief of the Journal of Neural Engineering.
CONTACT
W: nec.cwru.edu
FUNDING
Professor Durand’s work is funded through a Congressionally-Directed Medical Research Program grant, DARPA, the National Science Foundation, private donors, foundations, and the National Institutes of Health.
FURTHER READING
TE Eggers, YM Dweiri, GA McCallum, DM Durand, Recovering motor intent from chronic peripheral nerve recordings, Scientific reports, 2018, 8, 14149.
T Eggers, YM Dweiri, G McCallum, DM Durand, Model-based Bayesian signal extraction algorithm for peripheral nerves, Journal of Neural Engineering, 2017, 14, 056009.
YM Dweiri, TE Eggers, LE Gonzalez-Reyes, J Drain, GA McCallum, DM Durand, Stable detection of movement intent from peripheral nerves: Chronic study in dogs, Proceedings of IEEE, 2017, 105, 50–65, 2017.
Creative Commons Licence
(CC BY 4.0)
This work is licensed under a Creative Commons Attribution 4.0 International License.
What does this mean?
Share: You can copy and redistribute the material in any medium or format
Adapt: You can change, and build upon the material for any purpose, even commercially.
Credit: You must give appropriate credit, provide a link to the license, and indicate if changes were made.
More articles you may like
Dr Lifei Wang | Can Species Distribution Models Inform Us About Future Ecosystems?
The world is buzzing with news about how human activities and climate shifts are reshaping our ecosystems. Have you ever wondered how life will adapt to this rapidly changing world? Ecologists might be able to predict how different species will live in future using computer simulations. Dr Lifei Wang at the University of Toronto Scarborough investigates how different stimulations work under varying conditions to provide new insights into what may lie ahead.
Dr Yong Teng | Improving the Outlook for Head and Neck Cancer Patients
Dr Yong Teng at the Emory University School of Medicine is working with colleagues to overcome the high mortality of individuals diagnosed with cancers affecting the head and neck. One of his approaches is based on understanding the particular mechanisms of the ATAD3A gene, which new insights suggest are closely related to cancers affecting the head and neck.
Dr Tsun-Kong Sham – Dr Jiatang Chen – Dr Zou Finfrock – Dr Zhiqiang Wang | X-Rays Shine Light on Fuel Cell Catalysts
Understanding the electronic behaviour of fuel cell catalysts can be difficult using standard experimental techniques, although this knowledge is critical to their fine-tuning and optimisation. Dr Jiatang Chen at the University of Western Ontario works with colleagues to use the cutting-edge valence-to-core X-ray emission spectroscopy method to determine the precise electronic effects of altering the amounts of platinum and nickel in platinum-nickel catalysts used in fuel cells. Their research demonstrates the potential application of this technique to analysing battery materials, catalysts, and even cancer drug molecules.
Dr Michael Cherney – Professor Daniel Fisher | Unlocking Woolly Mammoth Mysteries: Tusks as Hormone Time Capsules
The impressive tusks found on proboscideans (the order of mammals that includes elephants, woolly mammoths, and mastodons) are like time capsules, preserving detailed records of their bearers’ lives in the form of growth layers and chemical traces. Frozen in time for thousands of years, these layers can unlock secrets about the lives of long-extinct relatives of modern elephants. Dr Michael Cherney and Professor Daniel Fisher from the University of Michigan used innovative techniques to extract and analyse steroid hormones preserved in woolly mammoth tusks. This ground-breaking work opens new avenues for exploring the biology and behaviour of extinct species.